User talk:Agaba Isaac
Conservation of linear momentum[edit]

The law of conservation of linear momentum is a fundamental law of nature, and it states that if no external force acts on a closed system of objects, the momentum of the closed system remains constant. One of the consequences of this is that the center of mass of any system of objects will always continue with the same velocity unless acted on by a force from outside the system.
Conservation of momentum is a mathematical consequence of the homogeneity (shift symmetry) of space (position in space is the canonical conjugate quantity to momentum). That is, conservation of momentum is equivalent to the fact that the physical laws do not depend on position.
In analytical mechanics the conservation of momentum is a consequence of translational invariance of Lagrangian in the absence of external forces. It can be proven that the total momentum is a constant of motion by making an infinitesimal translation of Lagrangian and then equating it with non translated Lagrangian. This is a special case of Noether's theorem .[1]
In an isolated system (one where external forces are absent) the total momentum will be constant: this is implied by Newton's first law of motion. Newton's third law of motion, the law of reciprocal actions, which dictates that the forces acting between systems are equal in magnitude, but opposite in sign, is due to the conservation of momentum.
Since position in space is a vector quantity, momentum (being the canonical conjugate of position) is a vector quantity as well—it has direction. Thus, when a gun is fired, the final total momentum of the system (the gun and the bullet) is the vector sum of the momenta of these two objects. Assuming that the gun and bullet were at rest prior to firing (meaning the initial momentum of the system was zero), the final total momentum must also equal 0.
In an isolated system with only two objects, the change in momentum of one object must be equal and opposite to the change in momentum of the other object. Mathematically,
Momentum has the special property that, in a closed system, it is always conserved, even in collisions and separations caused by explosive forces. Kinetic energy, on the other hand, is not conserved in collisions if they are inelastic. Since momentum is conserved it can be used to calculate an unknown velocity following a collision or a separation if all the other masses and velocities are known.
A common problem in physics that requires the use of this fact is the collision of two particles. Since momentum is always conserved, the sum of the momenta before the collision must equal the sum of the momenta after the collision:
where u1 and u2 are the velocities before collision, and v1 and v2 are the velocities after collision.
Determining the final velocities from the initial velocities (and vice versa) depend on the type of collision. There are two types of collisions that conserve momentum: elastic collisions, which also conserve kinetic energy, and inelastic collisions, which do not.
Elastic collisions[edit]

A collision between two pool balls is a good example of an almost totally elastic collision, due to their high rigidity; a totally elastic collision exists only in theory, occurring between bodies with mathematically infinite rigidity. In addition to momentum being conserved when the two balls collide, the sum of kinetic energy before a collision must equal the sum of kinetic energy after:
In one dimension[edit]
When the initial velocities are known, the final velocities for a head-on collision are given by

When the first body is much more massive than the other (that is, m1 » m2), the final velocities are approximately given by
Thus the more massive body does not change its velocity, and the less massive body travels at twice the velocity of the more massive body less its own original velocity. Assuming both masses were heading towards each other on impact, the less massive body is now therefore moving in the opposite direction at twice the speed of the more massive body plus its own original speed.
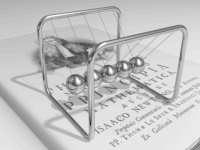
In a head-on collision between two bodies of equal mass (that is, m1 = m2), the final velocities are given by
Thus the bodies simply exchange velocities. If the first body has nonzero initial velocity u1 and the second body is at rest, then after collision the first body will be at rest and the second body will travel with velocity u1. This phenomenon is demonstrated by Newton's cradle.
In multiple dimensions[edit]
In the case of objects colliding in more than one dimension, as in oblique collisions, the velocity is resolved into orthogonal components with one component perpendicular to the plane of collision and the other component or components in the plane of collision. The velocity components in the plane of collision remain unchanged, while the velocity perpendicular to the plane of collision is calculated in the same way as the one-dimensional case.
For example, in a two-dimensional collision, the momenta can be resolved into x and y components. We can then calculate each component separately, and combine them to produce a vector result. The magnitude of this vector is the final momentum of the isolated system.
Perfectly inelastic collisions[edit]

A common example of a perfectly inelastic collision is when two snowballs collide and then stick together afterwards. This equation describes the conservation of momentum:
It can be shown that a perfectly inelastic collision is one in which the maximum amount of kinetic energy is converted into other forms. For instance, if both objects stick together after the collision and move with a final common velocity, one can always find a reference frame in which the objects are brought to rest by the collision and 100% of the kinetic energy is converted. This is true even in the relativistic case and utilized in particle accelerators to efficiently convert kinetic energy into new forms of mass-energy (i.e. to create massive particles).
Coefficient of restitution[edit]
The coefficient of restitution is defined as the ratio of relative velocity of separation to relative velocity of approach. It is a ratio hence it is a dimensionless quantity. The coefficient of restitution is given by:
for two colliding objects, where
- v1 is the scalar final velocity of the first object after impact,
- v2 is the scalar final velocity of the second object after impact,
- u1 is the scalar initial velocity of the first object before impact,
- u2 is the scalar initial velocity of the second object before impact.
A perfectly elastic collision implies that CR is 1. So the relative velocity of approach is same as the relative velocity of separation of the colliding bodies.
Inelastic collisions have (CR < 1). In case of a perfectly inelastic collision the relative velocity of separation of the centre of masses of the colliding bodies is 0. Hence the bodies stick together after collision.
Explosions[edit]
An explosion occurs as a result of a chain reaction that transforms potential energy into kinetic energy displacing the surrounding material. Explosions do not conserve potential energy. Instead, potential energy stored in chemical, mechanical, or nuclear form, is transformed into kinetic energy, acoustic energy, and electromagnetic radiation.
See the inelastic collision page for more details.