User:JS1451/sandbox
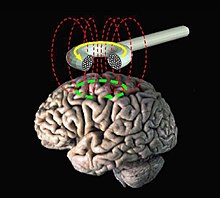
Motor Priming is the use of an excitatory or inhibitory stimulus prior to or in conjunction with the performance of a skilled movement in order to to affect performance, learning, or neurophysiological response[1][2][3]. Motor priming is derived from the psychological concept of priming, in which one stimulus unconsciously impacts response to a second stimulus[1][4].
Proposed Physiological Mechanisms
In most circumstances goal of motor priming is to use an initial or concurrent excitatory stimulus to depolarize neurons involved in motor pathways and reduce the activation threshold for subsequent stimuli[5][1]. This depolarization places the targeted neurons in a state of greater excitability, which is believed to motor learning through neuroplastic change[1][5]. Alternatively the use of inhibitory priming stimuli may be used to reduce interference from less functionally important neural pathways[1]. Initial inhibitory stimuli may also be used exploit homeostatic metaplasticity[6]. Proponents of this mechanism argue that plasticity brain is determined by the strength of the relevant stimulus relative to previous stimuli, rather than the absolute strength of the relevant single stimulus[6]. Thus, an inhibitory stimulus provided prior to training may lower the amount of activation required to induce neuroplastic change[6].
Forms of Motor Priming[edit]
Motor priming can be grouped into five major categories: stimulation-based priming, motor imagery and action observation, sensory priming, movement-based priming, and pharmacological priming[1].
Stimulation Based Priming[edit]

In stimulation based priming a non-invasive electrical or magnetic stimulus is administered to the nervous system with the intention of increasing movement dependent neuroplasticity[7][8]. The two most common forms of stimulation-based priming are transcranial magnetic stimulation (TMS) and transcranial direct current stimulation (tDCS). Together these techniques are referred to as non-invasive brain stimulation or NIBS as they stimulate targeted areas by generating electric or magnetic fields outside the skull[7][8]. Usually, NIBS targets the primary motor cortex or M1, however stimulation of other key movement related areas of the brain has been investigated[1].
Other forms of stimulation-based priming include peripheral nerve stimulation (PNS)[1] which functions in a similar manner to non-invasive brain stimulation, but targets a peripheral nerve[9], and paired associative stimulation (PAS)[1] which involves the concurrent provision of PNS and NIBS[10].
Motor Imagery and Action Observation[edit]
Motor imagery(or mental practice) involves the mental performance of a target task without the required physical movements, while action observation involves watching another individual perform the target activity in order to improve the performance of the observer[1][11]. Neuroimaging has shown activation in key motor areas in the brain during motor imagery and mental practice despite the lack of descending input to afferent peripheral nerves[12]. These forms of priming are thought to be most beneficial reducing physical workload for individuals with limited movement capacity and for allowing athletes a chance to prepare themselves for a skilled movement (i.e. a golfer may use motor imagery before attempting a difficult putt)[13][14].
Sensory Priming[edit]
Sensory priming involves the augmentation or reduction of external sensory stimuli with the intention of modulating motor performance[1][9]. Sensory augmentation techniques include, but are not limited to vibration, and peripheral electrical stimulation (which is considered a category of both sensory and stimulation-based priming)[1]. Vibration based techniques are generally applied to the antagonist muscle in an attempt to stimulate inhibitory neural pathways and reduce interference in agonist activities[1][15]. Peripheral nerve stimulation is generally applied to the nerve supplying the agonist muscle in order to enhance sensory feedback to the brain which in turn is thought to increase motor excitability[9].
Sensory deafferentation involves blocking of sensory signals from peripheral nerves generally using a tourniquet or anesthetic cream[1]. These techniques are have been applied to involved and uninvolved body areas with the intention of modulating neural plasticity[16][17]. A common target of sensory deafferentation is the contralateral extremity which is targeted to reduce interhemispheric inhibition[17].
Movement Based Priming[edit]
Movement based priming involves the performance of an additional motor task prior to practice of the target task[18]. There is a large amount of variability in movement based priming protocols, but they generally involve repetitive or continuous movement performed immediately prior to the target activity[18][1]. A common form of motor priming used in rehabilitation is bimanual which involves the performance of identical symmetric movements in the more and less effected limb[18]. This approach attempts to take advantage of the symmetry constraint or the propensity of toward symmetrical movements in both upper limbs[19].
Pharmacological Priming[edit]
In pharmacological priming drugs are provided with the intention of neuroplasticity during motor training[1]. The aim of these drugs is to upregulate excitatory signaling in the brain by either by directly increasing the quantity of excitatory neurotransmitters in the nervous system or by increasing the potency of such neurotransmitters by preventing their removal from the synaptic cleft[20][21]. Excitatory neurotransmitters targeted in in pharmacological priming include dopamine, norepinephrine, serotonin, and acetylcholine[1][20][21].
Use and Efficacy in Clinical Populations[edit]
In clinical settings, motor priming is most often used prior or in conjunction with to rehabilitative exercises in an attempt to facilitate recovery from neurological injury[1]. Due to variability in the parameters of priming techniques as well as differences in patient response due the variability of brain physiology at an individual level, results of individual motor priming studies have been mixed[22][23]. A 2020 metanalysis of 36 upper extremity motor priming techniques performed prior to task oriented training found moderate quality evidence of functional improvements due stimulation based priming and sensory priming[22]. The same metanalysis found low quality evidence of functional improvements due to movement-based priming, and inconclusive results for action observation[22]. A 2017 systematic review showed that non-invasive transcranial brain stimulation positively impacted motor function in individuals with Parkinson’s disease[23]. A 2007 systematic review found the use of amphetamines for motor priming no significant benefit in stroke[24]. The use of norepinephrine inhibitors following stroke has been supported by multiple studies[25]. A 2010 systematic review found that selective serotonin reuptake inhibitors(SSRIs) may improve motor recovery from stroke, but evidence was not strong enough to make recommendations for clinical practice[26].
Use and Efficacy in Non-clinical Populations[edit]
Motor priming has been studied in a diverse array of non-clinical contexts including but not limited to athletics and military training[27][28]. However, because there are fewer studies available for these populations more research is needed[27][28]. Like in clinical research motor priming studies targeting unimpaired individuals are subject to greatly variable results due to individual differences as well as differences in priming parameters[28]. The United States Airforce has performed multiple studies assessing the efficacy of non-invasive brain stimulation in improving cognitive and motor performance, but research in this area is still ongoing[29]. Noninvasive brain stimulation has also been used by athletes during training[28]. Early research in the use of priming techniques to improve athletic performance is promising, but there is less data available for these populations [28].
See also[edit]
References[edit]
- ^ a b c d e f g h i j k l m n o p q r s Stoykov, Mary Ellen; Madhavan, Sangeetha (2015-01). "Motor priming in neurorehabilitation". Journal of neurologic physical therapy: JNPT. 39 (1): 33–42. doi:10.1097/NPT.0000000000000065. ISSN 1557-0584. PMC 4270918. PMID 25415551.
{{cite journal}}
: Check date values in:|date=
(help) - ^ Stoykov, Mary Ellen; Corcos, Daniel Montie; Madhavan, Sangeetha (2017). "Movement-Based Priming: Clinical Applications and Neural Mechanisms". Journal of motor behavior. 49 (1): 88–97. doi:10.1080/00222895.2016.1250716. ISSN 0022-2895. PMC 6238643. PMID 28277966.
- ^ da Silva, Erika Shirley Moreira; Ocamoto, Gabriela Nagai; Santos-Maia, Gabriela Lopes Dos; de Fátima Carreira Moreira Padovez, Roberta; Trevisan, Claudia; de Noronha, Marcos Amaral; Pereira, Natalia Duarte; Borstad, Alexandra; Russo, Thiago Luiz (2020-06). "The Effect of Priming on Outcomes of Task-Oriented Training for the Upper Extremity in Chronic Stroke: A Systematic Review and Meta-analysis". Neurorehabilitation and Neural Repair. 34 (6): 479–504. doi:10.1177/1545968320912760. ISSN 1552-6844. PMID 32452242.
{{cite journal}}
: Check date values in:|date=
(help) - ^ Weingarten, Evan; Chen, Qijia; McAdams, Maxwell; Yi, Jessica; Hepler, Justin; Albarracin, Dolores (2016-5). "From Primed Concepts to Action: A Meta-Analysis of the Behavioral Effects of Incidentally-Presented Words". Psychological bulletin. 142 (5): 472–497. doi:10.1037/bul0000030. ISSN 0033-2909. PMC 5783538. PMID 26689090.
{{cite journal}}
: Check date values in:|date=
(help) - ^ a b Stinear, Cathy M.; Barber, P. Alan; Coxon, James P.; Fleming, Melanie K.; Byblow, Winston D. (2008-05). "Priming the motor system enhances the effects of upper limb therapy in chronic stroke". Brain: A Journal of Neurology. 131 (Pt 5): 1381–1390. doi:10.1093/brain/awn051. ISSN 1460-2156. PMID 18356189.
{{cite journal}}
: Check date values in:|date=
(help) - ^ a b c Ziemann, Ulf; Siebner, Hartwig R. (2008-01). "Modifying motor learning through gating and homeostatic metaplasticity". Brain Stimulation. 1 (1): 60–66. doi:10.1016/j.brs.2007.08.003. ISSN 1876-4754. PMID 20633369.
{{cite journal}}
: Check date values in:|date=
(help) - ^ a b Klomjai, Wanalee; Katz, Rose; Lackmy-Vallée, Alexandra (2015-09). "Basic principles of transcranial magnetic stimulation (TMS) and repetitive TMS (rTMS)". Annals of Physical and Rehabilitation Medicine. 58 (4): 208–213. doi:10.1016/j.rehab.2015.05.005. ISSN 1877-0665. PMID 26319963.
{{cite journal}}
: Check date values in:|date=
(help) - ^ a b Sparing, Roland; Mottaghy, Felix M. (2008-04). "Noninvasive brain stimulation with transcranial magnetic or direct current stimulation (TMS/tDCS)-From insights into human memory to therapy of its dysfunction". Methods (San Diego, Calif.). 44 (4): 329–337. doi:10.1016/j.ymeth.2007.02.001. ISSN 1046-2023. PMID 18374276.
{{cite journal}}
: Check date values in:|date=
(help) - ^ a b c Field-Fote, Edelle Carmen (2004-10). "Electrical stimulation modifies spinal and cortical neural circuitry". Exercise and Sport Sciences Reviews. 32 (4): 155–160. doi:10.1097/00003677-200410000-00006. ISSN 0091-6331. PMID 15604934.
{{cite journal}}
: Check date values in:|date=
(help) - ^ Carson, Richard G.; Kennedy, Niamh C. (2013-12-03). "Modulation of human corticospinal excitability by paired associative stimulation". Frontiers in Human Neuroscience. 7. doi:10.3389/fnhum.2013.00823. ISSN 1662-5161. PMC 3847812. PMID 24348369.
{{cite journal}}
: CS1 maint: unflagged free DOI (link) - ^ Barclay-Goddard, Ruth E.; Stevenson, Ted J.; Poluha, William; Thalman, Leyda (2011-05-11). "Mental practice for treating upper extremity deficits in individuals with hemiparesis after stroke". The Cochrane Database of Systematic Reviews (5): CD005950. doi:10.1002/14651858.CD005950.pub4. ISSN 1469-493X. PMC 6464751. PMID 21563146.
- ^ Decety, J.; Ingvar, D. H. (1990-02-XX). "Brain structures participating in mental simulation of motor behavior: a neuropsychological interpretation". Acta Psychologica. 73 (1): 13–34. doi:10.1016/0001-6918(90)90056-l. ISSN 0001-6918. PMID 2180254.
{{cite journal}}
: Check date values in:|date=
(help) - ^ Decety, J.; Ingvar, D. H. (1990-02-XX). "Brain structures participating in mental simulation of motor behavior: a neuropsychological interpretation". Acta Psychologica. 73 (1): 13–34. doi:10.1016/0001-6918(90)90056-l. ISSN 0001-6918. PMID 2180254.
{{cite journal}}
: Check date values in:|date=
(help) - ^ Malouin, Francine; Jackson, Philip L.; Richards, Carol L. (2013-09-19). "Towards the integration of mental practice in rehabilitation programs. A critical review". Frontiers in Human Neuroscience. 7: 576. doi:10.3389/fnhum.2013.00576. ISSN 1662-5161. PMC 3776942. PMID 24065903.
{{cite journal}}
: CS1 maint: unflagged free DOI (link) - ^ Liepert, Joachim; Binder, Christian (2010). "Vibration-induced effects in stroke patients with spastic hemiparesis--a pilot study". Restorative Neurology and Neuroscience. 28 (6): 729–735. doi:10.3233/RNN-2010-0541. ISSN 1878-3627. PMID 21209488.
- ^ Ziemann, Ulf; Hallett, Mark; Cohen, Leonardo G. (1998-09-01). "Mechanisms of Deafferentation-Induced Plasticity in Human Motor Cortex". The Journal of Neuroscience. 18 (17): 7000–7007. doi:10.1523/JNEUROSCI.18-17-07000.1998. ISSN 0270-6474. PMC 6792971. PMID 9712668.
- ^ a b Floel, Agnes; Hummel, Friedhelm; Duque, Julie; Knecht, Stefan; Cohen, Leonardo G. (2008). "Influence of Somatosensory Input on Interhemispheric Interactions in Patients With Chronic Stroke". Neurorehabilitation and neural repair. 22 (5): 477–485. doi:10.1177/1545968308316388. ISSN 1545-9683. PMC 4890542. PMID 18645188.
- ^ a b c Stinear, Cathy M.; Petoe, Matthew A.; Anwar, Samir; Barber, Peter Alan; Byblow, Winston D. (2014-01). "Bilateral priming accelerates recovery of upper limb function after stroke: a randomized controlled trial". Stroke. 45 (1): 205–210. doi:10.1161/STROKEAHA.113.003537. ISSN 1524-4628. PMID 24178916.
{{cite journal}}
: Check date values in:|date=
(help) - ^ Li, Yong; Levin, Oron; Carson, Richard G.; Swinnen, Stephan P. (2004-05). "Bimanual coordination: constraints imposed by the relative timing of homologous muscle activation". Experimental Brain Research. 156 (1): 27–38. doi:10.1007/s00221-003-1762-4. ISSN 0014-4819. PMID 14689135.
{{cite journal}}
: Check date values in:|date=
(help) - ^ a b Martinsson, L.; Hårdemark, H.; Eksborg, S. (2007-01-24). "Amphetamines for improving recovery after stroke". The Cochrane Database of Systematic Reviews (1): CD002090. doi:10.1002/14651858.CD002090.pub2. ISSN 1469-493X. PMID 17253474.
- ^ a b Mead, Gillian E.; Hsieh, Cheng-Fang; Lee, Rebecca; Kutlubaev, Mansur A.; Claxton, Anne; Hankey, Graeme J.; Hackett, Maree L. (2012-11-14). "Selective serotonin reuptake inhibitors (SSRIs) for stroke recovery". The Cochrane Database of Systematic Reviews. 11: CD009286. doi:10.1002/14651858.CD009286.pub2. ISSN 1469-493X. PMC 6465036. PMID 23152272.
- ^ a b c da Silva, Erika Shirley Moreira; Ocamoto, Gabriela Nagai; Santos-Maia, Gabriela Lopes Dos; de Fátima Carreira Moreira Padovez, Roberta; Trevisan, Claudia; de Noronha, Marcos Amaral; Pereira, Natalia Duarte; Borstad, Alexandra; Russo, Thiago Luiz (2020-06). "The Effect of Priming on Outcomes of Task-Oriented Training for the Upper Extremity in Chronic Stroke: A Systematic Review and Meta-analysis". Neurorehabilitation and Neural Repair. 34 (6): 479–504. doi:10.1177/1545968320912760. ISSN 1552-6844. PMID 32452242.
{{cite journal}}
: Check date values in:|date=
(help) - ^ a b Goodwill, Alicia M.; Lum, Jarrad A. G.; Hendy, Ashlee M.; Muthalib, Makii; Johnson, Liam; Albein-Urios, Natalia; Teo, Wei-Peng (2017-11-01). "Using non-invasive transcranial stimulation to improve motor and cognitive function in Parkinson's disease: a systematic review and meta-analysis". Scientific Reports. 7 (1): 14840. doi:10.1038/s41598-017-13260-z. ISSN 2045-2322. PMC 5665996. PMID 29093455.
- ^ Martinsson, L.; Hårdemark, H.; Eksborg, S. (2007-01-24). "Amphetamines for improving recovery after stroke". The Cochrane Database of Systematic Reviews (1): CD002090. doi:10.1002/14651858.CD002090.pub2. ISSN 1469-493X. PMID 17253474.
- ^ Foster, Donald J.; Good, David C.; Fowlkes, Allison; Sawaki, Lumy (2006-02). "Atomoxetine enhances a short-term model of plasticity in humans". Archives of Physical Medicine and Rehabilitation. 87 (2): 216–221. doi:10.1016/j.apmr.2005.08.131. ISSN 0003-9993. PMID 16442975.
{{cite journal}}
: Check date values in:|date=
(help) - ^ Mead, Gillian E.; Hsieh, Cheng-Fang; Lee, Rebecca; Kutlubaev, Mansur A.; Claxton, Anne; Hankey, Graeme J.; Hackett, Maree L. (2012-11-14). "Selective serotonin reuptake inhibitors (SSRIs) for stroke recovery". The Cochrane Database of Systematic Reviews. 11: CD009286. doi:10.1002/14651858.CD009286.pub2. ISSN 1469-493X. PMC 6465036. PMID 23152272.
- ^ a b Mason, Billy; McKune, Andrew; Pumpa, Kate; Ball, Nick (2020-11). "The Use of Acute Exercise Interventions as Game Day Priming Strategies to Improve Physical Performance and Athlete Readiness in Team-Sport Athletes: A Systematic Review". Sports Medicine (Auckland, N.Z.). 50 (11): 1943–1962. doi:10.1007/s40279-020-01329-1. ISSN 1179-2035. PMID 32779102.
{{cite journal}}
: Check date values in:|date=
(help) - ^ a b c d e Angius, Luca; Pascual-Leone, Alvaro; Santarnecchi, Emiliano (2018). "Brain stimulation and physical performance". Progress in Brain Research. 240: 317–339. doi:10.1016/bs.pbr.2018.07.010. ISSN 1875-7855. PMID 30390837.
- ^ Young, Emma. "Brain stimulation: The military's mind-zapping project". www.bbc.com. Retrieved 2021-04-25.
External links[edit]
- Research for this Wikipedia entry was conducted as a part of a Dr. Young-Hui Chang’s Locomotion Neuromechanics course (APPH 6232) offered in the School of Applied Physiology at Georgia Tech
- This page was greatly influenced by peer reviewed publications by Dr. Mary-Ellen Stoykov of Shirly Ryan Ability Lab and Northwestern University