User:Fizzelo/sandbox
Drug Treatment[edit]
Patients with thalassemia gradually accumulate high levels of iron (Fe) in their bodies. This build-up of iron may be due to the disease itself, from irregular haemoglobin not properly incorporating adequate iron into its structure, or it may be due to the many blood transfusions received by the patient. This overload of iron brings with it many biochemical complications.
Two key players involved in iron transport and storage in the body are ferritin and transferrin. Ferritin is a protein present within cells that binds to Fe (II) and stores it as Fe (III), releasing it into the blood whenever required. Transferrin is an iron-binding protein present in blood plasma; transferrin acts as a transporter, carrying iron through blood and providing cells with the metal through endocytosis. Transferrin is highly specific to iron (III), and binds to it with an equilibrium constant of 1023 M-1 at a pH of 7.4.[1]
Thalassemia results in nontransferrin-bound iron being available in blood as all the transferrin becomes fully saturated. This free iron is toxic to the body since it catalyzes reactions that generate free hydroxyl radicals.[2] These radicals may induce lipid peroxidation of organelles like lysosomes, mitochondria, and sarcoplasmic membranes. The resulting lipid peroxides may interact with other molecules to form cross links, and thus either cause these compounds to perform their functions poorly, or render them non-functional altogether.[2] This iron overload may be treated with chelation therapy. Deferoxamine, deferiprone and deferasirox are the three most widely-used iron-chelating agents.
Deferoxamine[edit]
Structure and Coordination[edit]
The drug deferoxamine, also known as desferoxamine B and DFO-B, is a trihydroxamic acid that is produced by the actinobacteria Streptomyces pilosus. It binds iron, decreasing the toxic reactions catalysed by the unbound metal, and it also decreases the uptake of iron by tissues. Deferoxamine achieves this by acting as a hexadentate iron-chelating ligand: it binds to all six coordination sites on nontransferrin-bound iron, effectively deactivating it.[3] Deferoxamine is mostly specific to ferric iron (Fe3+) and coordinates to Fe3+ using the oxygen atoms on its multiple hydroxyl and carbonyl groups, forming a structure called ferrioxamine. This drug-iron complex is mostly excreted by the kidneys as it is water-soluble.[4] Approximately one-third of ferrioxamine could also be excreted through the feces in bile.[2]
Administration and Action[edit]
Deferoxamine is administered via intravenous, intramuscular, or subcutaneous injections. Oral administration is not possible as deferoxamine is rapidly metabolized by enzymes and is poorly absorbed from the gastrointestinal tract. The required parenteral administration represents one of deferoxamine’s downfalls as it is harder for patients to follow up with their therapy due to the financial and emotional burdens experienced.[5] Deferoxamine was proven to cure many clinical complications and diseases that result from iron overload. It beneficially affects cardiac disease, such as myocardial disease which occurs as a result of iron accumulation in the heart.[6] Deferoxamine was also shown to improve liver function by arresting the development of hepatic fibrosis which occurs as a result of iron accumulation in the liver.[7] Deferoxamine also has positive effects on endocrine function and growth. Endocrine abnormalities in thalassemic patients involve the overloaded iron interfering with the production of insulin-like growth factor (IGF-1), as well as stimulating hypogonadism, both of which cause poor pubertal growth. A study showed that 90% of patients who were regularly treated with deferoxamine since childhood had normal pubertal growth, which fell to 38% for patients treated only with low doses of deferoxamine since their teens.[2] Another endocrine abnormality that thalassemic patients face is diabetes mellitus, which results from iron overload in the pancreas impairing insulin secretion. Studies have shown that patients who were regularly treated with deferoxamine have a reduced risk of developing diabetes mellitus.[8]
Side Effects[edit]
Deferoxamine could lead to toxic side effects if doses greater than 50 mg/kg body weight are administered. These side effects may include auditory and ocular abnormalities, pulmonary toxicity, sensorimotor neurotoxicity, as well as changes in renal function.[2] Another toxic effect of deferoxamine mostly observed in children is the failure of linear growth. This reduction in height may occur as a result of deferoxamine chelating metals other than iron which are required for normal growth. Deferoxamine has an affinity constant (Ka) of 1031 for Fe3+, 1014 for Cu2+ and 1010 for Zn2+, and so may coordinate to zinc and copper when little iron is available for chelation. Zinc is needed for the proper functioning of various metalloenzymes involved in bone formation. Zinc chelation may cause zinc deficiency in the body, which can thus lead to a reduced growth rate, reduced collagen formation and defective bone mineralization. Similarly, copper functions as an enzyme cofactor in bone formation. Copper chelation may result in copper deficiency as well, leading to metaphyseal cupping and osteoporosis. For example, abnormal collagen is formed when copper is deficient as the enzyme lysyl oxidase, which uses copper as a cofactor and catalyzes the oxidative deamination step that is important for cross-linking of collagen, cannot function properly. Studies have shown that even though the blood serum of patients receiving deferoxamine was not deficient in copper and zinc, deficiencies of the metals in the metaphyseal matrix were observed.
The toxic effect of deferoxamine on linear growth could also be due to excess deferoxamine accumulating in tissues and interfering with iron-dependent enzymes which are involved in the post-translational modification of collagen.[9]
Patients who receive vitamin C supplements have shown improved iron excretion by deferoxamine. This occurs due to the expansion of the iron pool brought about by vitamin C, which deferoxamine subsequently has access to. However, vitamin C supplementation could also worsen iron toxicity by promoting the formation of free radicals. Therefore, only 100 mg of vitamin C should be taken 30 minutes to one hour after deferoxamine administration.[10]
It has also been proven that combined treatment with deferoxamine and deferiprone leads to an increased efficiency in chelation and doubles iron excretion.[11]
Deferiprone[edit]
Structure and Coordination[edit]
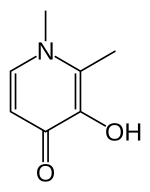
Deferiprone (DFP) is a bidentate iron-chelator. Three molecules of the drug therefore coordinate to one iron atom, forming an orthorhombic structure.[12][13]
DFP is synthetically made and is highly selective to Fe(III).[12][14] Physical properties that allow this compound to be effective as a drug include its water solubility, low molecular weight (139 Da), neutral charge, and lipophilicity.[12] These physio-chemical properties allow facile crossing of cell membranes throughout the body, including the blood-brain barrier, facilitating removal of excess iron from within organs.[12][15]
Although the mechanism for the removal of iron by DFP is not well understood, however, a study by Viroj Wiwanitkit in 2006 proposed a possible mechanism: the coordination to the iron was thought to occur through the cleavage of either a C-C bond or a C-O bond in the drug. Wiwanitkit concluded that the mechanism goes though the cleavage of the C-C bond because this bond requires less energy to be cleaved. The total energy for the cleavage was found to be negative, suggesting spontaneity and thermodynamic favourability of the cleavage. The resulting structure of the product also resembled the observed tertiary structure of the drug-iron complex.[13]
Administration and Action[edit]
Deferiprone is an iron chelator that is orally active, its administration thus being much easier than that for deferoxamine.[12] Plasma levels for the iron-drug complex climax after one hour of intake and the drug has a half-life of 160 minutes. Most of the iron-drug complex is therefore excreted within three to four hours following administration, the excretion occurring mostly in urine (90%).[12]
When comparing deferiprone to deferoxamine, it should be noted that they both bind iron with similar efficiency. However, drugs with different properties are able to access different iron pools. DFP is smaller than deferoxamine and can thus enter cells more easily. Also, at the pH of blood, the affinity of DFP for iron is concentration dependent: at low DFP concentrations, the iron-drug complex breaks down and the iron is donated to another competing ligand. This property accounts for the observed tendency of DFP to redistribute iron in the body. For the same reason, DFP can ‘shuttle’ intracellular iron out to the plasma, and transfer the iron to deferoxamine which goes on to expel it from the body.[14]
DFP was also found to be significantly more effective than deferoxamine in treating myocardial siderosis in patients with thalassemia major[12]: DFP is thought to improve the function of mitochondria in the heart by accessing and redistributing labile iron in cardiac cells.
Thalassemia patients may also be faced with potential oxidative damage to brain cells as the brain has high oxygen demands, but contains relatively low levels of antioxidant agents for protection against oxidation. The presence of excess iron in the brain may lead to higher concentrations of free radicals. Hexadentate chelators, like deferoxamine, are large molecules, and are thus unlikely to be able to cross the blood-brain barrier to chelate the excess iron. DFP, however, can do so and forms a soluble, neutral iron-drug complex that can cross cell membranes by non-facilitated diffusion. Attaching the drug to sugars may additionally enhance the penetration of the blood-brain barrier, as the brain uses facilitated transport for its relatively high levels of sugar intake.[16]
Side Effects[edit]
DFP can be subjected to glucuronidation in the liver, which may expel as much as 85% of the drug from the body before it has had a chance to chelate iron. DFP also has a well-known safety profile, with agranulocytosis being the most serious side effect.[12] While agranulocytosis has been reported in less than 2% of patients treated, it is potentially life threatening and thus requires close monitoring of the white blood cell count.[15] Less serious side effects include gastrointestinal symptoms, which were found in 33% of patients in the first year of administration, but fell to 3% in following years; arthralgia; and zinc deficiency, with the latter being a problem especially for individuals with diabetes.[12]
Deferasirox[edit]
Structure and Coordination[edit]

Deferasirox is an N-substituted bis-hydroxyphenyl-triazole. It is capable of removing iron from the blood through the coordination of two molecules of the deferasirox to a single iron ion, which forms the iron chelate (Fe-[deferasirox]2).[17] Each molecule of the tridentate chelator deferasirox binds to the iron at three sites, using one nitrogen atom and two oxygen atoms. This results in a stable octahedral geometry around the iron centre. The ability of deferasirox to remove iron stems directly from its relatively small size, which is what allows it to access the iron contained within the blood and, more notably, inside tissues. Also, an important feature of deferasirox is that it has been shown to be highly selective for iron in the +3 oxidation state, and use of the drug does not lead to a significant decrease in the levels of other important metals in the body.[18]
Administration and Action[edit]

Deferasirox is most commonly marketed under the brand name Exjade. It has one key advantage over desferoxamine in that it can be taken orally in pill form, and so does not require intravenous or subcutaneous administration. With a terminal elimination half life of 8-16 hours, the deferasirox pill can be taken just once everyday. A once-daily dose of 20mg/kg of body weight has been found to be sufficient for most patients for the maintenance of liver iron concentration (LIC) levels, which are usually measured as mg of iron per g of liver tissue. Larger doses may be required for some patients in order to reduce LIC levels.[19] The ability of deferasirox to effectively reduce LIC levels has been well documented. One study demonstrated that after 4-5 years of deferasirox treatment the mean LIC levels of patients decreased from 17.4 ± 10.5 to 9.6 ± 8.0 mg Fe/g. This study showed that long-term treatment did result in a sustainable reduction in the iron burden faced by patients receiving blood transfusions for thalassemia.[20] An additional benefit of the use of deferasirox instead of desferoxamine is that, unlike desferoxamine, early studies have indicated that deferasirox does not have a significant impact on the growth and development of pediatric thalassemia patients. In a study by Cappellini et. al. it was shown that children receiving the treatment displayed continual near-normal growth and development over a 5-year study period.[20]
Side Effects[edit]
Deferoxamine can, however, have a wide variety of side effects. These may include headaches, nausea, vomiting, and joint pains.[21] Some evidence has been shown of a link to gastrointestinal disorders experienced by some people who have received the treatment.[20]
Indicaxanthin[edit]
Structure[edit]

Indicaxanthin is a pigment derived from the cactus pear fruit and can be used as an antioxidant. Dietary indicaxanthin has been shown to have protective effects on RBCs in people with beta thalassemia.[22] It has a structure similar to that of amino acids, and is amphiphilic: it is able to bind to cell membranes through charge-related interactions with polar head groups of membrane constituents, as well through adsorption to the lipid aggregates. Upon ex vivo introduction to thalassemic blood, indicaxanthin was shown to accumulate within RBCs.[22]
Function[edit]
Hb undergoes the following oxidation reaction during normal controlled breakdown of RBCs:
Hb → Oxy-Hb → Met-Hb → [Perferryl-Hb] → Oxoferryl → further oxidation steps
This reaction is experienced by thalassemic RBCs to a greater extent because, not only are there more oxidative radicals in thalassemic blood, but thalassemic RBCs also have limited antioxidant defense. Indicaxanthin is able to reduce the perferryl-Hb, a reactive intermediate, back to met-Hb. The overall effect of this step is that Hb degradation is prevented, which helps prevent accelerated breakdown of RBCs.[22]
In addition, indicaxathin has been shown to reduce oxidative damage in cells and tissues and does so by binding to radicals. The mechanism of its function, however, is still unknown.[22]
Indicaxanthin has high bioavailability and minimal side effects, like vomiting or diarrhea.
STAT5[edit]
Signal Transducer and Activator of Transcription 5 refers to two highly related proteins, STAT5A and STAT5B, which are part of the seven-membered STAT family of proteins. Though STAT5A and STAT5B are encoded by separate genes, the proteins are 90% identical at the amino acid level.[23] STAT5 proteins are involved in cytosolic signalling and in mediating the expression of specific genes.[24] Aberrant STAT5 activity has been shown to be closely connected to a wide range of human cancers,[25] and silencing this aberrant activity is an area of active research in medicinal chemistry.[26]
Activation and Action[edit]
In order to be functional, STAT5 proteins must first be activated. This activation is carried out by kinases associated with transmembrane receptors[25]:
- first, ligands binding to these transmembrane receptors on the outside of the cell activate the kinases;
- second, the stimulated kinases add a phosphate group to a specific tyrosine residue on the receptor;
Domains of STAT proteins - STAT5 then binds to these phosphorylated-tyrosines using their SH2 domain (STAT domains illustrated to the right);
- the bound STAT5 is then phosphorylated by the kinase, the phosphorylation occurring at particular tyrosine residues on the C-terminus of the protein
- phosphorylation causes STAT5 to dissociate from the receptor

- the phosphorylated STAT5 finally goes on to form either homodimers, STAT5-STAT5, or heterodimers, STAT5-STATX, with other STAT proteins. The SH2 domains of the STAT5 proteins are once again used for this dimerization.
The activation pathway is illustrated to the left, where the ligand involved is a cytokine and the specific kinase taking part in activation is JAK. The dimerized STAT5 represents the active form of the protein, which is ready for translocation into the nucleus.
Once in the nucleus, the dimers bind to STAT5 response elements, inducing transcription of specific sets of genes. Upregulation of gene expression by STAT5 dimers has been observed for genes dealing with[24]:
- controlled cell growth and division, or cell proliferation
- programmed cell death, or apoptosis
- cell specialization, or differentiation and
- inflammation.
Activated STAT5 dimers are, however, short-lived and the dimers are made to undergo rapid deactivation. Deactivation may be carried out by a direct pathway, removing the phosphate groups using phosphatases like PIAS or SHP-2 for example, or by an indirect pathway, which involves reducing cytokine signalling.[27]
STAT5 and cancer[edit]
STAT5 has been found to be constitutively phosphorylated in cancer cells[26], implying that the protein is always present in its active form. This constant activation is brought about either by mutations or by aberrant expressions of cell signalling, resulting in poor regulation, or complete lack of control, of the activation of transcription for genes influenced by STAT5. This leads to constant and increased expression of these genes. For example, mutations may lead to increased expression of anti-apoptotic genes, the products of which actively prevent cell death. The constant presence of these products preserve the cell in spite of it having become cancerous, causing the cell to eventually become malignant.
Treatment approaches[edit]
Attempts at treatment for cancer cells with constitutively phosphorylated STAT5 have included both indirect and direct inhibition of STAT5 activity. While more medicinal work has been done in indirect inhibition, this approach can lead to increased toxicity in cells and can also result in non-specific effects, both of which are better handled by direct inhibition.[26]
Indirect inhibition targets kinases associated with STAT5, or targets proteases that carry out terminal truncation of proteins. Different inhibitors have been designed to target different kinases:
- inhibition of BCR/ABl constitutes the basis of the functioning of drugs like imatinib[28] and PD180790[29]
- inhibition of FLT3 is carried out by drugs like lestaurtinib[30] and sorafenib[31]
- inhibition of JAK2 is carried out by the drug CYT387, which was successful in preclinical trials and is currently undergoing clinical trials.[32]
Direct inhibition of STAT5 activity makes use of small molecule inhibitors that prevent STAT5 from properly binding to DNA, or prevent proper dimerization. The inhibiting of DNA binding utilizes RNA interference,[33] antisense oligodeoxynucleotide,[33] and short hairpin RNA.[34] The inhibition of proper dimerization, on the other hand, is brought about by the use of small molecules that target the SH2 domain. Recent work on drug development in the latter field have proved particularly effective.[35]
References[edit]
- ^ Aisen P, Leibman A, Zweier J (March 1978). "Stoichiometric and site characteristics of the binding of iron to human transferrin". J. Biol. Chem. 253 (6): 1930–7. doi:10.1016/S0021-9258(19)62337-9. PMID 204636.
{{cite journal}}
: CS1 maint: date and year (link) CS1 maint: multiple names: authors list (link) - ^ a b c d e >Olivieri, N. F.; Brittenham, G. M. (1997). "Iron-chelating therapy and the treatment of thalassemia". Journal of the American Society of Hematology. 89 (3): 739–761. PMID 9028304. Retrieved 28 February 2013.
{{cite journal}}
: CS1 maint: date and year (link) - ^ Griffith, Patricia M. (1994). "Efficacy of deferoxamine in preventing complications of iron overload in patients with thalassemia major". The New England Journal of Medicine. 331 (9): 567–573. doi:10.1056/NEJM199409013310902. PMID 8047080. ProQuest 223989174. Retrieved March 5, 2013.
- ^ Cozar, O.; Leopold, N.; Jelic, C.; Chiş, V.; David, L.; Mocanu, A.; Tomoaia-Cotişel, M. (2006). "IR, Raman and surface-enhanced Raman study of desferrioxamine B and its Fe(III) complex, ferrioxamine B." Journal of Molecular Structure. 788 (1–3): 1–6. doi:10.1016/j.molstruc.2005.04.035. Retrieved March 1, 2013.
{{cite journal}}
: CS1 maint: date and year (link) - ^ Cohen, Alan; Martin, Marie; Schwartz, Elias (1981). "Response to long-term deferoxamine therapy in thalassemia". The Journal of Pediatrics. 99 (5): 689–694. doi:10.1016/S0022-3476(81)80385-X. PMID 7299539. Retrieved March 2, 2013.
{{cite journal}}
: CS1 maint: date and year (link) - ^ Wonke, Beatrix (2005). "Randomized controlled trial of deferiprone or deferoxamine in beta-thalassemia major patients with asymptomatic myocardial siderosis". Journal of the American Society of Hematology. 107: 3738–3744. Retrieved March 2, 2013.
{{cite journal}}
: Unknown parameter|coauthors=
ignored (|author=
suggested) (help) - ^ Jin, Haiyan; Terai, Shuji; Sakaida, Isao (2007). "The iron chelator deferoxamine causes activated hepatic stellate cells to become quiescent and to undergo apoptosis" (PDF). Journal of Gastroenterology. 42 (6): 475–484. doi:10.1007/s00535-007-2020-5. PMID 17671763. S2CID 34700148. Retrieved March 3, 2013.
{{cite journal}}
: CS1 maint: date and year (link) - ^ Kaye, Todd B.; Guay, André T.; Simonson, Donald C. (1993). "Non-insulin-dependent diabetes mellitus and elevated serum ferritin level". Journal of Diabetes and Its Complications. 7 (4): 246. doi:10.1016/S0002-9610(05)80252-1. Retrieved March 5, 2013.
{{cite journal}}
: CS1 maint: date and year (link) - ^ Olivieri, Nancy F.; Koren, Gideon; Harris, Jonathan; Khattak, Sohail; Freedman, Melvin H.; Templeton, Douglas M.; Bailey, John D.; Reilly, B. J. (1992). "Growth failure and bony changes induced by deferoxamine". American Journal of Pediatric Hematology/Oncology. 14 (1): 48–56. doi:10.1097/00043426-199221000-00007. PMID 1550263. Retrieved March 5, 2013.
{{cite journal}}
: CS1 maint: date and year (link) - ^ Ambruso, D. R.; Mahony, B. S.; Githens, J. H.; Rhoades, E. D. (1982). "Effect of subcutaneous deferoxamine and oral vitamin C on iron excretion in congenital hypoplastic anemia and refractory anemia associated with the 5q-syndrome". The American Journal of Pediatric Hematology/Oncology. 4 (2): 115–123. PMID 7114394. Retrieved March 10, 2013.
{{cite journal}}
: CS1 maint: date and year (link) - ^ Kattamis, Antonis (2005). "Combined therapy with deferoxamine and deferiprone". Annals of the New York Academy of Sciences. 1054: 175–182. doi:10.1196/annals.1345.020. PMID 16339663. S2CID 29566007. Retrieved March 10, 2013.
- ^ a b c d e f g h i Berdoukas, Vasili; Farmaki; Carson; Wood; Coates (2012). "Treating Thalassemia Major-Related Iron Overload: The Role of Deferiprone". Journal of Blood Medicine. 3: 119–129. doi:10.2147/JBM.S27400. PMC 3480237. PMID 23112580.
{{cite journal}}
: CS1 maint: date and year (link) CS1 maint: unflagged free DOI (link) - ^ a b Wiwanitkit, Viroj (2006). "Quantum Chemical Analysis of the Deferiprone-Iron Binding Reaction". International Journal of Nanomedicine. 1 (1): 111–113. doi:10.2147/nano.2006.1.1.111. PMC 2426763. PMID 17722270.
{{cite journal}}
: CS1 maint: unflagged free DOI (link) - ^ a b Olivieri, Nancy F.; Brittenham, Gary M. (1997). "Iron-Chelating Therapy and the Treatment of Thalassemia". Blood. 89 (3): 739–761. doi:10.1182/blood.V89.3.739. PMID 9028304.
{{cite journal}}
: CS1 maint: date and year (link) - ^ a b Galanello, R.; Campus, S. (2009). "Deferiprone Chelation Therapy for Thalassemia Major". Acta Haematologica. 122 (2–3): 155–164. doi:10.1159/000243800. PMID 19907153. S2CID 31161087.
{{cite journal}}
: CS1 maint: date and year (link) - ^ Heli, H.; Mirtorabi, S.; Karimian, K. (2011). "Advances in iron chelation: an update". Expert Opinion on Therapeutic Patents. 21 (6): 819–856. doi:10.1517/13543776.2011.569493. PMID 21449664. S2CID 41639654.
{{cite journal}}
: CS1 maint: date and year (link) - ^ Cappellini, M. D.; Cohen, A.; Piga, A.; Bejaoui, M.; Perrotta, S.; Agaoglu, L.; Aydinok, Y.; Kattamis, A.; Kilinc, Y.; Porter, J.; Capra, M.; Galanello, R.; Fattoum, S.; Drelichman, G.; Magnano, C.; Verissimo, M.; Athanassiou-Metaxa, M.; Giardina, P.; Kourakli-Symeonidis, A.; Janka-Schaub, G.; Coates, T.; Vermylen, C.; Olivieri, N.; Thuret, I.; Opitz, H.; Ressayre-Djaffer, C.; Marks, P.; Alberti, D. (2006). "Aphase 3 study of deferasirox (ICL670), a once-daily oral iron chelator, in patients with -thalassemia". Blood. 107 (9): 3455–3462. doi:10.1182/blood-2005-08-3430. PMID 16352812.
{{cite journal}}
: CS1 maint: date and year (link) - ^ Galanello; et al. (2006). "Phase II clinical evaluation of deferasirox, a once-daily oral chelating agent, in pediatric patients with β-thalassemia major". Haematologica. 91 (10): 1343–1351. PMID 17018383.
{{cite journal}}
: Explicit use of et al. in:|last=
(help) - ^ Nisbet-Brown, Eric; Olivieri, Nancy F.; Giardina, Patricia J.; Grady, Robert W.; Neufeld, Ellis J.; Séchaud, Romain; Krebs-Brown, Axel J.; Anderson, Judith R.; Alberti, Daniele; Sizer, Kurt C.; Nathan, David G. (2003). "Effectiveness and safety of ICL670 in iron-loaded patients with thalassemia: a randomised, doubleblind, placebo-controlled, dose-escalation trial". Lancet. 361 (9369): 1597–1602. doi:10.1016/S0140-6736(03)13309-0. PMID 12747879. S2CID 12396225.
{{cite journal}}
: CS1 maint: date and year (link) - ^ a b c Cappellini, M. Domenica; Bejaoui, Mohamed; Agaoglu, Leyla; Canatan, Duran; Capra, Marcello; Cohen, Alan; Drelichman, Guillermo; Economou, Marina; Fattoum, Slaheddine; Kattamis, Antonis; Kilinc, Yurdanur; Perrotta, Silverio; Piga, Antonio; Porter, John B.; Griffel, Louis; Dong, Victor; Clark, Joan; Aydinok, Yesim (31). "Iron chelation with deferasirox in adult and pediatric patients with thalassemia major: efficacy and safety during 5 years' follow-up". Blood. 118 (4): 884–893. doi:10.1182/blood-2010-11-316646. PMID 21628399. S2CID 17856195.
{{cite journal}}
: Check date values in:|date=
and|year=
/|date=
mismatch (help); Unknown parameter|month=
ignored (help) - ^ "How Are Thalassemias Treated?". National Heat, Lung and Blood institute. Retrieved March 2, 2013.
- ^ a b c d Tesoriere, L.; Allegra, M.; Butera, D.; Gentile, C.; Livrea, M. A. (July 2006). "Cytoprotective effects of the antioxidant phytochemical indicaxanthin in beta-thalassemia red blood cells". Free Radical Research. 40 (7): 753–761. doi:10.1080/10715760600554228. PMID 16984002. S2CID 25837703.
{{cite journal}}
: CS1 maint: date and year (link) - ^ Grimley PM, Dong F, Rui H (June 1999). "Stat5a and Stat5b: fraternal twins of signal transduction and transcriptional activation". Cytokine Growth Factor Rev. 10 (2): 131–57. doi:10.1016/S1359-6101(99)00011-8. PMID 10743504.
{{cite journal}}
: CS1 maint: date and year (link) CS1 maint: multiple names: authors list (link) - ^ a b Nosaka, T.; Kawashima, T.; Misawa, K.; Ikuta, K.; Mui, A. L.; Kitamura, T. (1999). "STAT5 as a molecular regulator of proliferation, differentiation and apoptosis in hematopoietic cells". EMBO J. 18 (17): 4754–4765. doi:10.1093/emboj/18.17.4754. PMC 1171548. PMID 10469654.
{{cite journal}}
: CS1 maint: date and year (link) - ^ a b Tan, S.-H.; Nevalainen, M. T. (2008). "Signal transducer and activator of transcription 5A/B in prostate and breast cancers". Endocr. Relat. Cancer. 15 (2): 367–390. doi:10.1677/ERC-08-0013. PMC 6036917. PMID 18508994.
{{cite journal}}
: CS1 maint: date and year (link) - ^ a b c Cumaraswamy, Abbarna A.; Todic, Aleksandra; Resetca, Diana; Minden, Mark D.; Gunning, Patrick T. (2012). "Inhibitors of Stat5 protein signalling". Med. Chem. Commun. 3: 22–27. doi:10.1039/C1MD00175B.
{{cite journal}}
: CS1 maint: date and year (link) - ^ Shuai, K.; Halpern, J.; Ten Hoeve, J.; Rao, X.; Sawyers, C. L. (1996). "Constitutive activation of STAT5 by the BCR-ABL oncogene in chronic myelogenous leukemia". Oncogene. 13 (2): 247–254. PMID 8710363.
{{cite journal}}
: CS1 maint: date and year (link) - ^ Druker, Brian J.; Tamura, Shu; Buchdunger, Elisabeth; Ohno, Sayuri; Segal, Gerald M.; Fanning, Shane; Zimmermann, Jürg; Lydon, Nicholas B. (1996). "Effects of a selective inhibitor of the Abl tyrosine kinase on the growth of BCR-Abl positive cells". Nat. Med. 2 (5): 561–566. doi:10.1038/nm0596-561. PMID 8616716. S2CID 36102747.
{{cite journal}}
: CS1 maint: date and year (link) - ^ Huang, Mei; Dorsey, Jay F.; Epling-Burnette, PK; Nimmanapalli, Ramadevi; Landowski, Terry H.; Mora, Linda B.; Niu, Guilian; Sinibaldi, Dominic; Bai, Fanqi; Kraker, Alan; Yu, Hua; Moscinski, Lynn; Wei, Sheng; Djeu, Julie; Dalton, William S.; Bhalla, Kapil; Loughran, Thomas P.; Wu, Jie; Jove, Richard (2002). "Inhibition of BCR-Abl kinase activity by PD180970 blocks constitutive activation of Stat5 and growth of CML cells". Oncogene. 21 (57): 8804–8816. doi:10.1038/sj.onc.1206028. PMID 12483533. S2CID 24650286.
{{cite journal}}
: CS1 maint: date and year (link) - ^ Levis, Mark; Allebach, Jeffrey; Tse, Kam-Fai; Zheng, Rui; Baldwin, Brenda R.; Smith, B. Douglas; Jones-Bolin, Susan; Ruggeri, Bruce; Dionne, Craig; Small, Donald (2002). "A FLT3-targeted tyrosine kinase inhibitor is cytotoxic to leukemia cells in vitro and in vivo". Blood. 99 (11): 3885–3891. doi:10.1182/blood.V99.11.3885. PMID 12010785.
{{cite journal}}
: CS1 maint: date and year (link) - ^ Auclair, D.; Miller, D.; Yatsula, V.; Pickett, W.; Carter, C.; Chang, Y.; Zhang, X.; Wilkie, D.; Burd, A.; Shi, H.; Rocks, S.; Gedrich, R.; Abriola, L.; Vasavada, H.; Lynch, M.; Dumas, J.; Trail, P. A.; Wilhelm, S. M. (2007). "Antitumor activity of sorafenib in FLT3-driven leukemic cells". Leukemia. 21 (3): 439–445. doi:10.1038/sj.leu.2404508. PMID 17205056. S2CID 21261134.
{{cite journal}}
: CS1 maint: date and year (link) - ^ Pardanani, A.; Lasho, T.; Smith, G.; Burns, C. J.; Fantino, E.; Tefferi, A. (2009). "CYT387, a selective JAK1/JAK2 inhibitor: In vitro assessment of kinase selectivity and preclinical studies using cell lines and primary cells from polycythemia vera patients". Leukemia. 23 (8): 1441–1445. doi:10.1038/leu.2009.50. PMID 19295546. S2CID 26947444.
{{cite journal}}
: CS1 maint: date and year (link) - ^ a b Behbod, Fariba; Nagy, Zsuzsanna S.; Stepkowski, Stanislaw M.; Karras, James; Johnson, Charlene R.; Jarvis, W. David; Kirken, Robert A. (2003). "Specific Inhibition of Stat5a/B Promotes Apoptosis of IL-2-Responsive Primary and Tumor-Derived Lymphoid Cells". J Immunol. 171 (8): 3919–3927. doi:10.4049/jimmunol.171.8.3919. PMID 14530308. S2CID 7713780.
{{cite journal}}
: CS1 maint: date and year (link) - ^ Klosek, S. K.; Nakashiro, K.; Hara, S.; Goda, H.; Hamakawa, H. (2008). "Stat3 as a molecular target in RNA interference-based treatment of oral squamous cell carcinoma". Oncol Rep. 20 (4): 873–878. PMID 18813829.
{{cite journal}}
: CS1 maint: date and year (link) - ^ Gunning, P. T. (2011). J Med Chem.
{{cite journal}}
: Missing or empty|title=
(help); Unknown parameter|coauthors=
ignored (|author=
suggested) (help)