User:Cooper rachel/sandbox
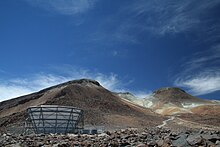

The Atacama Cosmology Telescope (ACT) is a six meter telescope on Cerro Toco in the Atacama Desert in the north of Chile, near the Llano de Chajnantor Observatory. It is designed to make high-resolution, microwave-wavelength surveys of the sky in order to study the cosmic microwave background radiation (CMB). The primary goals of the project are to measure primordial fluctuations in the early universe, detect galaxy clusters using the Sunyaev-Zel'dovich effect (SZ), conclusively observe gravitational lensing, and detect new star-forming galaxies.
Erected in the first half of 2007, ACT saw first light on 22 October 2007 with its initial science receiver, the Millimeter Bolometer Array Camera (MBAC). ACT completed three seasons of observing with the MBAC detector in 2010. In 2013, ACT took its first observations with ACTPol, an upgraded polarized detector.
The project is a collaboration between Princeton University, the University of Pennsylvania, NASA/GSFC, the University of British Columbia, NIST, the Pontificia Universidad Católica de Chile, the University of KwaZulu-Natal, Cardiff University, Rutgers University, the University of Pittsburgh, Haverford College, INAOE, the University of Toronto, the University of Massachusetts Amherst . It is funded by the US National Science Foundation and supported by the National Commission for Scientific and Technological Research (CONICYT) in Chile.
The South Pole Telescope has made complementary observations with similar science objectives.
Location[edit]
Ideal conditions for the location of a millimeter-wavelength telescope are high-altitude, arid conditions. Water vapor in the atmosphere can both absorb and emit radiation at these wavelengths, creating atmospheric signals which can be much larger than astronomical signals. ACT benefits from its location in the lofty—yet easily accessible—Chajnantor plain in the Andean mountains in the Atacama Desert. At an altitude of 5190 meters (17,030 feet), it is one of the highest permanent, ground-based telescopes in the world.[1] Several other observatories are located in the region, including CBI, ASTE, Nanten, APEX and ALMA.
Optical Design[edit]
ACT is an off-axis Gregorian telescope, with a six meter (236 in) primary mirror and a two meter (79 in) secondary mirror. Both mirrors are ellipsoid segments and consist of 71 (primary) and 11 (secondary) aluminum panels. Unlike most telescopes which track the rotating sky during observation, ACT observes a strip of sky, typically five degrees wide, by scanning back and forth in azimuth at the relatively rapid rate of two degrees per second. The rotating portion of the telescope weighs approximately 32 tonnes (35 short tons), creating a substantial engineering challenge. A ground screen surrounding the telescope minimizes contamination from microwave radiation emitted by the ground. Nearby ambient-temperature objects, which could load the sensors with radiation, also pose a risk of data error. The design of ACT combats this with reflective aluminum "guard rings" around each mirror. These rings expand the area of the mirrors beyond the field of the camera so that all information reaching the sensors comes from the sky. The design, manufacture and construction of the telescope were done by Dynamic Structures in Vancouver, British Columbia.
The MBAC receiver used refractive rather than reflective optics. The full receiver array contained three separate optics tubes, each containing a set of cold lenses, one for each of the three frequency bands at which the MBAC detects microwaves. The MBAC lenses are made out of silicone with a high refractive index to minimize attenuation. Also included in each optics tube are various interference filters including band-pass filters, low-pass capacitive mesh filters, and infrared filters. The first lens, cooled to 1K, forms the image from the primary mirror onto the next cold lens. The second and third lenses refocus the image onto the MBAC focal plane. This entire set of optics is contained in a light-tight tube, all cooled to 0.3K, because any thermal radiation emission past the lenses reaches the sensors without further filtering.
The current ACTPol receiver employs a similar optical design. The radiation is imaged in two linear polarizations by ADD STUFF HERE. ACTPol also uses novel machined surface features, rather than a coating, to reduce reflection from the lenses. REF
Detectors[edit]
ACT detects microwave radiation using arrays of silicon transition edge sensing bolometers. For the MBAC, radiation was detected in each of three frequency bands centered at 148 GHz, 218 GHz and 277 GHz. Each 3 cm x 3 cm (1.2" x 1.2") array has 1024 detectors, for a total of 3072 detectors. A system of cryogenic helium refrigerators kept the MBAC detectors cooled to a third of a degree above absolute zero (0.3K). An improved refrigerator design for ACTPol results in detector temperatures of around 0.1K.
Each detector is held at its superconducting transition temperature by an applied voltage. Small changes in the detector temperature due to slight differences in absorbed radiation result in large changes in the electrical resistance of the detector because it is at the superconducting transition.
The resulting sensitivity is sufficient to measure the temperature of the microwave background radiation to within a few millionths of a degree.[2]
Sky Maps[edit]
The ACT collaboration has published maps LINK of around 1000 square degrees of the microwave sky in two frequencies (148 GHz and 218 GHz). The maps have angular resolutions of 1.4 arcminutes (148 GHz) and 1.1 arcminutes (218 GHz). The noise level in the maps ranges from 30 to 50 micro-Kelvin CHECK for the average signal in each square arcminute. One region is a strip along the celestial equator, and a second region is a patch in the southern sky at a declination of -55 degrees. The equatorial strip overlaps many other astronomical surveys in a range of frequency bands, including the Stripe 82 region of the Sloan Digital Sky Survey. LINK
ACT measures the signal from each of its detectors 40 times each second. CHECK This data time stream, together with information about the pointing direction of the telescope at each time, is used to construct a best-estimate map of the sky, using maximum likelihood statistical techniques. REFERENCE The resulting maps are the experiment's fundamental scientific product, and have then been used to investigate a range of topics in astronomy and cosmology.
Results[edit]
ACT released results measuring the statistical properties of the CMB temperature variations down to arcminute angular scales in January 2010.[3] It found signals that were consistent with unresolved point sources and the Sunyaev-Zeldovich effect. In 2011, ACT made the first detection of the power spectrum of gravitational lensing of the microwave background,[1] which, combined with the WMAP satellite results on larger angular scales, provided evidence for dark energy from the CMB alone.[4] ACT has also discovered many new galaxy clusters from their thermal SZ signal, and galaxy cluster motions via their kinematic SZ signal.
Power Spectrum[edit]
Power spectra describe the distribution of microwave background temperature fluctuations over angular scales. ACT produced temperature power spectra using three seasons of data (2008, 2009, and 2010) which covered approximately 600 square degrees of the sky at the 148 GHz and 218 GHz frequencies. The power spectra range over angular scales from 0.35 degrees to a little over one arcminute. The angular scale of these temperature fluctuations allows us to constrain the basic model of the early universe. Numerous null tests demonstrate that any systematic error in the ACT power spectra are below the threshold of statistical error. The ACT power spectrum data are consistent with those from the overlapping regions mapped by the South Pole Telescope (SPT) and the Wilkinson Microwave Anisotropy Probe (WMAP) 9-year data.
These data tightly constrain the primary parameters of the standard Lambda-CDM model of cosmology, in addition to some secondary parameters. The ACT data is consistent with the data from SPT and WMAP.[5]
Sunyaev-Zel'dovich Effect[edit]
The Sunyaev–Zel'dovich (SZ) effect is a distortion in the CMB in which high-energy electrons give a slight energy boost to lower-energy CMB photons through the process of Compton scattering. This distorts the blackbody spectrum of the CMB, and makes it possible to detect galaxy clusters via their hot gas, which distorts the spectrum of the radiation passing through them.
The ACT collaboration has reported 68 galaxy clusters, 19 of which are new discoveries, detected via the tSZ effect at the 148 GHz frequency in a surveyed area of 504 square degrees centered around the celestial equator, ranging in redshift from approximately 0.1 to 1.4. 270 square degrees of the surveyed area overlaps with Sloan Digital Sky Survey Stripe 82, and is estimated to be 90% complete.[6] Clusters are detected by temperature decrements in the 148 GHz maps at cluster locations. The distribution of ACT cluster masses and redshifts are consistent with the standard model of cosmology.
There are currently relatively few SZ-detected clusters as compared to those detected using optical or X-ray technologies [ref], but many of the most massive and distant clusters have been discovered via their SZ signal. Several studies [ref] have noted that the discovery of a very massive cluster at high redshift would challenge the ΛCDM model. Since the SZ signal from a cluster is largely independent of the cluster redshift, this technique is well-suited to find such clusters if they exist.
El Gordo[edit]
The ACT collaboration discovered a particularly extreme galaxy cluster, known as El Gordo (ACT-CL J0102-4915), through its particularly strong thermal SZ signal. It was subsequently confirmed by optical and X-ray data. At redshift 0.87, it is "likely the most massive, hottest, most X-ray luminous and brightest Sunyaev-Zel'dovich (SZ) effect cluster known at redshifts greater than 0.6," according to ACT collaborator Felipe Menanteau. It is less than 5% likely that a cluster at such a high redshift would be found at all under the parameters of the ΛCDM model, let alone in the small percentage of sky mapped by the ACT. However, the existence of El Gordo does not pose a threat to the ΛCDM model, because it falls in the lower mass range statistically allowed under the parameters of the model. It is unlikely that more than approximately three such massive clusters would have been created at such an early stage of the evolution of the universe, according to the currently accepted model of the universe. Sunyaev-Zel'dovich, optical (dynamical), and X-ray masses were calculated for El Gordo. Though these values range over a factor of two, they are statistically consistent, and the combined mass estimates come to 2.16±0.32, with the stellar content <1% of the total mass. These values were corroborated by SPT data [ref]. Merging cluster systems at high redshifts, such as El Gordo, provide ideal settings for several astrophysical topics. Their different initial and final conditions allow research into dark matter and cluster assembly through such cluster merges. Other interesting features of El Gordo will provide opportunities for ongoing research. Further mapping will allow confirmation of an offset between baryonic and dark matter mass distribution as well as spatial temperature distribution within the cluster. Interesting features ripe for further investigation include an area of X-ray brightness indicative of a wake of hot gas resulting from the passage of one cluster through another, and the gas distribution of this wake implies a low-density cavity within the cluster. Possible future studies will look at the evolution of this feature, the speed of the merger, and the relative masses and velocities of the clusters.[7]
Cluster Movement[edit]
ACT confirmed for the first time the motion of galaxy clusters by means of the kinematic Sunyaev-Zel'dovich effect (kSZ). In this subset of the SZ effect, the CMB photons interact with the electrons in the galaxy clusters, causing the microwaves moving through these clusters to appear hotter or colder, depending on the direction of the galaxy's motion. Because the magnitude of the kSZ signal is independent of the distance of the cluster from Earth, temperature variations indicative of movement can be observed even in extremely distant clusters. The theory that moving galaxy clusters would distort the temperature of the CMB was first developed in 1972 by Sunyaev and Zel'dovich [ref], but ACT's recent work is the first confirmation of the kSZ effect.
ACT worked together with the Baryon Oscillation Spectroscopic Survey (BOSS), a part of the Sloan Digital Sky Survey III, to compile data which would not have been conclusive on its own. Data from 27,291 galaxies from BOSS were overlaid with ACT survey maps of the same sky region to confirm the temperature fluctuations that indicate cluster motion.[8]
The discovery was named on of Physics World magazine's top 10 breakthroughs for 2012.
Gravitational Lensing[edit]
Gravitational lensing, the distribution of matter capable of bending light as it travels towards the observer, was first detected in the cosmic microwave background by ACT. Other studies have found evidence of lensing by other means, but ACT first detected it using only the temperature maps to create a four-point convergence power spectrum. The effect is not visible to the naked eye in the maps, but is statistically apparent. Possible sources of data contamination, such as point sources and SZ clusters, were filtered out. 480 simulated versions of the ACT temperature maps were analyzed to create an average power spectrum, which, when compared to the actual power spectrum, was consistent enough to confirm the data. The amplitude of the lensing signal was consistent with the theoretical signal under the basic model.[9]
Both Physics Today and Science News magazines listed this discovery as one of the top physics stories of 2011 [ref].
Point Sources[edit]
Point sources in astrophysics are sources of radiation with such small angular dimensions that their extent appears negligible in maps of electromagnetic emissions of the universe. In the case of ACT, objects less than one arcminute (1/60 degree) appear as point sources. The 2008 ACT observing season maps at 148 GHz, which covered an area of 455 square degrees, were used for detection and analysis of 157 point sources.
Extragalactic point sources that appear in ACT's maps at 148 GHz are usually one of two types: active galactic nuclei (AGN) or "dusty galaxies." The spectra emitted by AGN consist of synchrotron radiation extending towards low frequencies, while those of dusty galaxies have low emissivity (graybody radiation) increasing towards submillimeter frequencies. The radiation from the AGN comes from mass accretion in the super-massive black holes at the center of the galaxies. The radiation from dusty galaxies is believed to be emitted by dust from rapid star formation.
Understanding point source emission is critical for the interpretation of anistropies in the CMB as well as the SZ signal from galaxy clusters. Point sources are a significant part of the overall brightness of the sky, and such bright foreground signals must be able to be subtracted from the background radiation in order for accurate conclusions to be drawn about several cosmological theories. CMB measurements at small angular scales constrain the primordial fluctuations of the universe. At even smaller scales, studies of the CMB are attempting to discern between contributions of the SZ effect and the fluctuations of the matter density field. To constrain the form of the SZ spectrum, an even more in-depth understanding of the energy from AGN and dusty galaxies is necessary. Surveys of the SZ effect looking for dark energy and other cosmological parameters will also need to know more about the behavior of point sources to avoid data biases.
The ACT catalog of point sources shows a 98% consistency with other source catalogs at lower frequencies, including the SPT and WMAP7 data at 30 GHz, among others.[10]
South Pole Telescope[edit]
Measurements of the CMB power spectrum from the South Pole Telescope were subsequently released [11] that were later also shown to provide evidence for dark energy from the CMB alone.[12]
See also[edit]

References[edit]
- ^ a b The Receiver Lab Telescope (RLT), an 80 cm instrument, is higher at 5525 meters, but is not permanent as it is fixed to the roof of a movable shipping container. Marrone (2005). "Observations in the 1.3 and 1.5 THz Atmospheric Windows with the Receiver Lab Telescope". Sixteenth International Symposium on Space Terahertz Technology: 64. arXiv:astro-ph/0505273. Bibcode:2005stt..conf...64M.
{{cite journal}}
: Unknown parameter|coauthors=
ignored (|author=
suggested) (help) The new University of Tokyo Atacama Observatory is signicantly higher than both. Cite error: The named reference ":0" was defined multiple times with different content (see the help page). - ^ Fowler, J. W.; Niemack, M. D.; Dicker, S. R.; Aboobaker, A. M.; Ade, P. A. R.; Battistelli, E. S.; Devlin, M. J.; Fisher, R. P.; Halpern, M.; Hargrave, P. C.; Hincks, A. D.; Kaul, M.; Klein, J.; Lau, J. M.; Limon, M.; Marriage, T. A.; Mauskopf, P. D.; Page, L.; Staggs, S. T.; Swetz, D. S.; Switzer, E. R.; Thornton, R. J.; Tucker, C. E. (2007). "Optical Design of the Atacama Cosmology Telescope and the Millimeter Bolometric Array Camera". Applied Optics. 46 (17): 3444–54. arXiv:astro-ph/0701020. Bibcode:2007ApOpt..46.3444F. doi:10.1364/AO.46.003444. PMID 17514303.
{{cite journal}}
: CS1 maint: date and year (link) - ^ Fowler, J. W.; et al. (2010). "The Atacama Cosmology Telescope: A Measurement of the 600 < >>>ℓ < 8000 Cosmic Microwave Background Power Spectrum at 148 GHz". The Astrophysical Journal. 722 (2): 1148–1161. arXiv:1001.2934. Bibcode:2010ApJ...722.1148F. doi:10.1088/0004-637X/722/2/1148.
{{cite journal}}
: CS1 maint: date and year (link) - ^ Sherwin, Blake D.; et al. (2011). "The Atacama Cosmology Telescope: Detection of the Power Spectrum of Gravitational Lensing". Physical Review Letters. 107 (2): 021302. arXiv:1105.0419. Bibcode:2011PhRvL.107b1302S. doi:10.1103/PhysRevLett.107.021302. PMID 21797591.
{{cite journal}}
: CS1 maint: date and year (link) - ^ Sievers, J. L.; et al. (ACT Collaboration) (2013). "The Atacama Cosmology Telescope: Cosmological Parameters From Three Seasons Of Data". arXiv:1301.0824
- ^ Hasselfield, M.; et al. (ACT collaboration) (2013). "The Atacama Cosmology Telescope: Sunyaev-Zel'dovich Selected Galaxy Clusters at 148 GHz from Three Seasons of Data". arXiv:1301.0816
- ^ Menanteau, F.; et al. (ACT Collaboration) (2012). "The Atacama Cosmology Telescope: ACT-CL J0102-4915 'El Gordo,' a Massive Merging Cluster at Redshift 0.87". arXiv:1109.0953
- ^ Hand, N.; et al. (ACT Collaboration) (2012). "Evidence of Galaxy Cluster Motions with the Kinematic Sunyaev-Zel'dovich Effect". arXiv:1203.4219
- ^ Das, S.; et al. (ACT Collaboration)(2011)."Detection of the Power Spectrum of Cosmic Microwave Background Lensingby the Atacama Cosmology Telescope". arXiv:1103.2124
- ^ Marriage, T.A.; et al. (The ACT Collaboration) (2011). "The Atacama Cosmology Telescope: Extragalactic Sources at 148 GHz in the 2008 Survey". arXiv:1007.5256
- ^ Keisler, R.; et al. (2011). "A Measurement of the Damping Tail of the Cosmic Microwave Background Power Spectrum with the South Pole Telescope". Astrophysical Journal. 743 (1): 28. arXiv:1105.3182. Bibcode:2011ApJ...743...28K. doi:10.1088/0004-637X/743/1/28.
{{cite journal}}
: CS1 maint: date and year (link) - ^ Van Engelen, A.; et al. (2012). "A measurement of gravitational lensing of the microwave background using South Pole Telescope data". Astrophysical Journal. 756 (2): 142. arXiv:1202.0545. Bibcode:2012ApJ...756..142V. doi:10.1088/0004-637X/756/2/142.
{{cite journal}}
: CS1 maint: date and year (link)
External links[edit]
22°57′31″S 67°47′16″W / 22.95861°S 67.78778°W
Category:Cosmic microwave background experiments Category:Submillimetre telescopes Category:Astronomical observatories in Chile Category:Buildings and structures in Antofagasta Region