User:E.Thorsos/sandbox
DRAFT UPDATE TO 'POLYCYCLIC AROMATIC HYDROCARBON'
Sources & distribution[edit]
Polycyclic aromatic hydrocarbons are primarily produced from the incomplete combustion of organic matter. PAHs can also be produced geologically when organic sediments are chemically transformed into fossil fuels such as oil and coal as a result of increased pressure and temperature in the sedimentary layer.[1] The dominant sources of PAHs in the environment are thus from human activity: Wood-burning and combustion of other biofuels such as dung or crop residues contribute more than half of annual global PAH emissions, particularly due to biofuel use in India and China.[2] As of 2004, industrial processes and the extraction and use of fossil fuels made up slightly more than one quarter of global PAH emissions, dominating outputs in industrial countries such as the United States.[2] Wild fires are another notable source.[2] Substantially higher outdoor air, soil, and water concentrations of PAHs have been measured in Asia, Africa, and Latin America than in Europe, Australia, and the U.S./Canada.[2]
PAHs are typically generated in complex mixtures, and the exact composition of a PAH mixture depends on the source.[1][3] Lower-temperature combustion, such as tobacco smoking or wood-burning, tends to generate low molecular weight PAHs, whereas high-temperature industrial processes typically generate PAHs with higher molecular weights.[3]
Human exposure[edit]
Human exposure varies across the globe and depends on factors such as smoking rates, fuel types in cooking, and pollution controls on power plants, industrial processes, and vehicles.[1][2][4] Developed countries with stricter air and water pollution controls, cleaner sources of cooking (i.e., gas and electricity vs. coal or biofuels), and prohibitions of public smoking tend to have lower levels of PAH exposure, while developing and undeveloped countries tend to have higher levels.[1][2][4]
Burning solid fuels such as coal and biofuels in the home for cooking and heating is a dominant global source of PAH emissions that in developing countries leads to high levels of exposure to indoor particulate air pollution containing PAHs, particularly for women and children who spend more time in the home or cooking.[2][5]
In industrial countries, people who smoke tobacco products, or who are exposed to second-hand smoke, are among the most highly exposed groups; tobacco smoke contributes to 90% of indoor PAH levels in the homes of smokers.[4] For the general population in developed countries, the diet is otherwise the dominant source of PAH exposure, particularly from smoking or grilling meat or consuming PAHs deposited on plant foods, especially broad-leafed vegetables, during growth.[6] PAHs are typically at low concentrations in drinking water.[4]

Emissions from vehicles such as cars and trucks can be a substantial outdoor source of PAHs in particulate air pollution.[1][2] Geographically, major roadways are thus sources of PAHs, which may distribute in the atmosphere or deposit nearby.[7] Catalytic converters are estimated to reduce PAH emissions from gasoline-fired vehicles by 25-fold.[1]
People can also be occupationally exposed during work that involves fossil fuels or their derivatives, wood burning, carbon electrodes, or exposure to diesel exhaust.[8][9] Industrial activity that can produce and distribute PAHs includes aluminum, iron, and steel manufacturing; coal gasification, tar distillation, shale oil extraction; production of coke, creosote, carbon black, and calcium carbide; road paving and asphalt manufacturing; rubber tire production; manufacturing or use of metal working fluids; and activity of coal or natural gas power stations.[1][8][9]
Environmental distribution & degradation[edit]
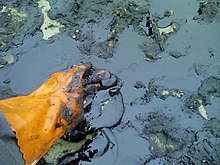
PAHs typically disperse from urban and suburban non-point sources through road run-off, sewage, and atmospheric circulation and subsequent deposition of particulate air pollution.[10] Soil and river sediment near industrial sites such as creosote manufacturing facilities can be highly contaminated with PAHs.[1] Oil spills, creosote, coal mining dust, and other fossil fuel sources can also distribute PAHs in the environment.[1][11]
Two- and three-ring PAHs can disperse widely while dissolved in water or as gases in the atmosphere, while PAHs with higher molecular weights can disperse locally or regionally adhered to particulate matter that is suspended in air or water until the particles land or settle out of the water column.[1] PAHs have a strong affinity for organic carbon, and thus highly organic sediments in rivers, lakes, and the ocean can be a substantial sink for PAHs.[7]
Algae and some invertebrates such as protozoans, mollusks, and many polychaetes have limited ability to metabolize PAHs and bioaccumulate disproportionate concentrations of PAHs in their tissues; however, PAH metabolism can vary substantially across invertebrate species.[10][12] Most vertebrates metabolize and excrete PAHs relatively rapidly.[10] Tissue concentrations of PAHs do not increase (biomagnify) from the lowest to highest levels of food chains.[10]
PAHs transform slowly to a wide range of degradation products. Biological degradation by microbes is a dominant form of PAH transformation in the environment.[13][14] Soil-consuming invertebrates such as earthworms speed PAH degradation, either through direct metabolism or by improving the conditions for microbial transformations.[14] Non-biological transformation products in the atmosphere and the top layers of surface waters can include nitrogenated, halogenated, hydroxylated, and oxygenated PAHs; some of these compounds can be more toxic, water soluble, and mobile than their parent PAHs.[10][15][16]
Minor sources[edit]
Volcanic eruptions may emit PAHs.[1] Certain PAHs such as perylene can also be generated in anaerobic sediments from existing organic material, although it remains undetermined whether abiotic or microbial processes drive their production.[17][18][19]
Human health[edit]
Cancer is a primary human health risk of exposure to PAHs.[20] Exposure to PAHs has also been linked with cardiovascular disease and poor fetal development.
Cancer[edit]
PAHs have been linked to skin, lung, bladder, liver, and stomach cancers in well-established animal model studies.[20] Specific compounds classified by various agencies as possible or probable human carcinogens are identified in the section "Regulation and Oversight" below.
Historical significance[edit]
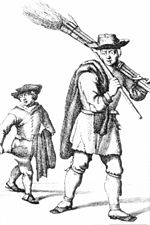
Historically, PAHs contributed substantially to our understanding of adverse health effects from exposures to environmental contaminants, including chemical carcinogenesis.[21] In 1775, Percivall Pott, a surgeon at St. Bartholomew’s Hospital in London, observed that scrotal cancer was unusually common in chimney sweepers and proposed the cause as occupational exposure to soot.[22] A century later, Richard von Volkmann reported increased skin cancers in workers of the coal tar industry of Germany, and by the early 1900s increased rates of cancer from exposure to soot and coal tar was widely accepted. In 1915, Yamigawa and Ichicawa were the first to experimentally produce cancers, specifically of the skin, by topically applying coal tar to rabbit ears.[22]
In 1922, Ernest Kennaway determined that the carcinogenic component of coal tar mixtures was an organic compound consisting of only C and H. This component was later linked to a characteristic fluorescent pattern that was similar but not identical to benz[a]anthracene, a PAH that was subsequently demonstrated to cause tumors.[22] Cook, Hewett and Hieger then linked the specific spectroscopic fluorescent profile of benzo[a]pyrene to that of the carcinogenic component of coal tar,[22] the first time that a specific compound from an environmental mixture (coal tar) was demonstrated to be carcinogenic.
In the 1930s and later, epidemiologists from Japan, England, and the U.S., including Richard Doll and various others, reported greater rates of death from lung cancer following occupational exposure to PAH-rich environments among workers in coke ovens and coal carbonization and gasification processes.[23]
Mechanisms of carcinogenesis[edit]

The structure of a PAH influences whether and how the individual compound is carcinogenic.[20][24] Some carcinogenic PAHs are genotoxic and induce mutations that initiate cancer; others are not genotoxic and instead affect cancer promotion or progression.[24][25]
PAHs that affect cancer initiation are typically first chemically modified by enzymes into metabolites that react with DNA, leading to mutations. When the DNA sequence is altered in genes that regulate cell replication, cancer can result. Mutagenic PAHs, such as benzo[a]pyrene, usually have four or more aromatic rings as well as a "bay region", a structural pocket that increases reactivity of the molecule to the metabolizing enzymes.[26] Mutagenic metabolites of PAHs include diol epoxides, quinones, and radical PAH cations.[27][26][28] These metabolites can bind to DNA at specific sites, forming bulky complexes called DNA adducts that can be stable or unstable.[22][29] Stable adducts may lead to DNA replication errors, while unstable adducts react with the DNA strand, removing a purine base (either adenine or guanine).[29] Such mutations, if they are not repaired, can transform genes encoding for normal cell signaling proteins into cancer-causing oncogenes.[24] Quinones can also repeatedly generate reactive oxygen species that may independently damage DNA.[26]
Enzymes in the cytochrome family (CYP1A1, CYP1A2, CYP1B1) metabolize PAHs to diol epoxides.[30] PAH exposure can increase production of the cytochrome enzymes, allowing the enzymes to convert PAHs into mutagenic diol epoxides at greater rates.[30] In this pathway, PAH molecules bind to the aryl hydrocarbon receptor (AhR) and activate it as a transcription factor that increases production of the cytochrome enzymes. The activity of these enzymes may at times conversely protect against PAH toxicity, which is not yet well understood.[30]
Low molecular weight PAHs, with 2 to 4 aromatic hydrocarbon rings, are more potent as co-carcinogens during the promotional stage of cancer. In this stage, an initiated cell (i.e., a cell that has retained a carcinogenic mutation in a key gene related to cell replication) is removed from growth-suppressing signals from its neighboring cells and begins to clonally replicate.[31] Low molecular weight PAHs that have bay or bay-like regions can dysregulate gap junction channels, interfering with intercellular communication, and also affect mitogen-activated protein kinases that activate transcription factors involved in cell proliferation.[31] Closure of gap junction protein channels is a normal precursor to cell division. Excessive closure of these channels after exposure to PAHs results in removing a cell from the normal growth-regulating signals imposed by its local community of cells, thus allowing initiated cancerous cells to replicate. These PAHs do not need to be enzymatically metabolized first. Low molecular weight PAHs are prevalent in the environment, thus posing a significant risk to human health at the promotional phases of cancer.
Cardiovascular disease[edit]
Adult exposure to PAHs has been linked to cardiovascular disease.[32] PAHs are among the complex suite of contaminants in cigarette smoke and particulate air pollution and may contribute to cardiovascular disease resulting from such exposures.[33]
In laboratory experiments, animals exposed to certain PAHs have shown increased development of plaques (atherogenesis) within arteries.[34] Potential mechanisms for the pathogenesis and development of atherosclerotic plaques may be similar to the mechanisms involved in the carcinogenic and mutagenic properties of PAHs.[34] A leading hypothesis is that PAHs may activate the cytochrome enzyme CYP1B1 in vascular smooth muscle cells. This enzyme then metabolically processes the PAHs to quinone metabolites that bind to DNA in reactive adducts that remove purine bases. The resulting mutations may contribute to unregulated growth of vascular smooth muscle cells or to their migration to the inside of the artery, which are steps in plaque formation.[33][34] These quinone metabolites also generate reactive oxygen species that may alter the activity of genes that affect plaque formation.[34]
Oxidative stress following PAH exposure could also result in cardiovascular disease by causing inflammation, which has been recognized as an important factor in the development of atherosclerosis and cardiovascular disease.[35][36] Biomarkers of exposure to PAHs in humans have been associated with inflammatory biomarkers that are recognized as important predictors of cardiovascular disease, suggesting that oxidative stress resulting from exposure to PAHs may be a mechanism of cardiovascular disease in humans.[37]
Developmental impacts[edit]
Multiple epidemiological studies of people living in Europe, the United States, and China have linked in utero exposure to PAHs, through air pollution or parental occupational exposure, with poor fetal growth, reduced immune function, and poorer neurological development, including lower IQ.[38][39][40][41]
Ecotoxicology[edit]
Wildlife exposed to environmental PAHs may develop many of the same health problems that humans face from PAH exposure, including cancer and effects on reproduction and development. Researchers have also observed that fish exposed to PAHs can exhibit heart deformities and impaired immune function.[42][43]
Heart deformities[edit]
In fish and mammals, chronic exposure to certain PAHs during embryo development induces severe, fatal heart deformities and other problems in the cardiovascular system. In rivers highly polluted with PAHs by industrial sites, populations of the Atlantic mummichog (Fundulus heteroclitus) have rapidly evolved the ability to tolerate PAH exposure without developing heart deformities.[42]
The mechanisms by which PAHs are developmentally toxic to the cardiovascular system likely vary with PAH structure.[42] Small three-ring PAHs such as fluorene and phenanthrene may be directly toxic to the developing cardiovascular system. Some larger PAHs likely disrupt development by binding to and activating the aryl hydrocarbon receptor, which then increases production of cytochrome enzymes or other unknown proteins that affect development. However, some larger PAHs that activate the aryl hydrocarbon receptor do not lead to developmental toxicity, demonstrating that these molecular pathways are complex and that whether and how individual PAHs affect the physical development of vertebrates can vary substantially. Oxidative stress after metabolic enzymes convert PAHs to more reactive compounds likely also plays a role.[42]
Immune system[edit]
PAHs appear to be immunotoxic in both fish and mammals, with effects on both specific and non-specific immunity.[43] Fish from environments that are highly contaminated with PAHs have symptoms consistent with suppressed immune function, including a higher rate of infections. These immunotoxic effects may develop only after cytochrome enzymes metabolize the PAHs to more reactive compounds. PAHs also appear to increase Ca2+ concentrations in phagocytes and lymphocytes, which may inhibit these cells' proliferation during the immune response.[43]
References[edit]
- ^ a b c d e f g h i j k Ravindra, K; Sokhi, R; Van Grieken, R (2008). "Atmospheric polycyclic aromatic hydrocarbons: Source attribution, emission factors and regulation". Atmospheric Environment. 42 (13): 2895–2921. doi:10.1016/j.atmosenv.2007.12.010. hdl:2299/1986. ISSN 1352-2310. Retrieved 2014-08-12.
- ^ a b c d e f g h Ramesh, A.; Archibong, A.; Hood, D.B.; Guo, Z.; Loganathan, B. G. (2011). "Global environmental distribution and human health effects of polycyclic aromatic hydrocarbons". Global Contamination Trends of Persistent Organic Chemicals. Boca Raton, FL: CRC Press. pp. 97–126. ISBN 978-1-4398-3831-0.
- ^ a b Tobiszewski, M; Namieśnik, J (2012). "PAH diagnostic ratios for the identification of pollution emission sources". Environmental Pollution. 162: 110–119. doi:10.1016/j.envpol.2011.10.025. ISSN 0269-7491. PMID 22243855. Retrieved 2015-05-12.
- ^ a b c d Choi, H.; Harrison, R.; Komulainen, H.; Delgado Saborit, J. (2010). "Polycyclic aromatic hydrocarbons". WHO Guidelines for Indoor Air Quality: Selected Pollutants. Geneva: World Health Organization. Retrieved 2014-12-12.
- ^ Kim, K-H; Jahan, S A; Kabir, E (2011). "A review of diseases associated with household air pollution due to the use of biomass fuels". Journal of Hazardous Materials. 192 (2): 425–431. doi:10.1016/j.jhazmat.2011.05.087. ISSN 0304-3894. PMID 21705140. Retrieved 2015-12-11.
- ^ Phillips, D H (1999). "Polycyclic aromatic hydrocarbons in the diet". Mutation Research/Genetic Toxicology and Environmental Mutagenesis. 443 (1–2): 139–147. doi:10.1016/S1383-5742(99)00016-2. ISSN 1383-5718. PMID 10415437. Retrieved 2015-02-05.
- ^ a b Srogi, K. (2007). "Monitoring of environmental exposure to polycyclic aromatic hydrocarbons: a review". Environmental Chemistry Letters. 5 (4): 169–195. doi:10.1007/s10311-007-0095-0. ISSN 1610-3661. PMC 5614912. PMID 29033701.
- ^ a b Boffetta, P; Jourenkova, N; Gustavsson, P (1997). "Cancer risk from occupational and environmental exposure to polycyclic aromatic hydrocarbons". Cancer Causes & Control. 8 (3): 444–472. doi:10.1023/A:1018465507029. ISSN 1573-7225. PMID 9498904. S2CID 35174373. Retrieved 2014-12-03.
- ^ a b Wagner, M; Bolm-Audorff, U; Hegewald, J; Fishta, A; Schlattmann, P; Schmitt, J; Seidler, A (2015). "Occupational polycyclic aromatic hydrocarbon exposure and risk of larynx cancer: a systematic review and meta-analysis". Occupational and Environmental Medicine. 72 (3): 226–233. doi:10.1136/oemed-2014-102317. ISSN 1470-7926. PMID 25398415. S2CID 25991349. Retrieved 2015-04-13.
- ^ a b c d e Hylland, K (2006). "Polycyclic aromatic hydrocarbon (PAH) ecotoxicology in marine ecosystems". Journal of Toxicology and Environmental Health, Part A. 69 (1–2): 109–123. doi:10.1080/15287390500259327. ISSN 1528-7394. PMID 16291565. S2CID 23704718. Retrieved 2015-04-02.
- ^ Achten, C.; Hofmann, T. (2009). "Native polycyclic aromatic hydrocarbons (PAH) in coals – A hardly recognized source of environmental contamination". Science of the Total Environment. 407 (8): 2461–2473. doi:10.1016/j.scitotenv.2008.12.008. ISSN 0048-9697. PMID 19195680. Retrieved 2015-04-09.
- ^ Jørgensen, A; Giessing, A M. B.; Rasmussen, L J; Andersen, O (2008). "Biotransformation of polycyclic aromatic hydrocarbons in marine polychaetes". Marine Environmental Research. 65 (2): 171–186. doi:10.1016/j.marenvres.2007.10.001. ISSN 0141-1136. PMID 18023473. S2CID 6404851. Retrieved 2016-01-22.
- ^ Haritash, A. K.; Kaushik, C. P. (2009). "Biodegradation aspects of polycyclic aromatic hydrocarbons (PAHs): A review". Journal of Hazardous Materials. 169 (1–3): 1–15. doi:10.1016/j.jhazmat.2009.03.137. ISSN 0304-3894. PMID 19442441. Retrieved 2014-08-20.
- ^ a b Johnsen, A. R.; Wick, L. Y.; Harms, H. (2005). "Principles of microbial PAH-degradation in soil". Environmental Pollution. 133 (1): 71–84. doi:10.1016/j.envpol.2004.04.015. ISSN 0269-7491. PMID 15327858. Retrieved 2015-05-07.
- ^ Lundstedt, S.; White, P. A.; Lemieux, C. L.; Lynes, K. D.; Lambert, I. B.; Öberg, L.; Haglund, P.; Tysklind, M. (2007). "Sources, fate, and toxic hazards of oxygenated polycyclic aromatic hydrocarbons (PAHs) at PAH- contaminated sites". AMBIO: A Journal of the Human Environment. 36 (6): 475–485. doi:10.1579/0044-7447(2007)36[475:SFATHO]2.0.CO;2. ISSN 0044-7447. PMID 17985702. S2CID 36295655. Retrieved 2015-04-09.
- ^ Fu, P. P.; Xia, Q; Sun, X; Yu, H (2012). "Phototoxicity and Environmental Transformation of Polycyclic Aromatic Hydrocarbons (PAHs)—Light-Induced Reactive Oxygen Species, Lipid Peroxidation, and DNA Damage". Journal of Environmental Science and Health, Part C. 30 (1): 1–41. doi:10.1080/10590501.2012.653887. ISSN 1059-0501. PMID 22458855. S2CID 205722865. Retrieved 2015-12-11.
- ^ Meyers, P. A.; Ishiwatari, R. (1993). "Lacustrine organic geochemistry—an overview of indicators of organic matter sources and diagenesis in lake sediments". Organic Geochemistry. 20 (7): 867–900. doi:10.1016/0146-6380(93)90100-P. hdl:2027.42/30617. ISSN 0146-6380. Retrieved 2015-02-04.
- ^ Silliman, J E; Meyers, P A; Eadie, B J; Val Klump, J (2001). "A hypothesis for the origin of perylene based on its low abundance in sediments of Green Bay, Wisconsin". Chemical Geology. 177 (3–4): 309–322. doi:10.1016/S0009-2541(00)00415-0. ISSN 0009-2541. Retrieved 2015-02-04.
- ^ Wakeham, S. G.; Schaffner, C.; Giger, W. (1980). "Poly cyclic aromatic hydrocarbons in Recent lake sediments—II. Compounds derived from biogenic precursors during early diagenesis". Geochimica et Cosmochimica Acta. 44 (3): 415–429. doi:10.1016/0016-7037(80)90041-1. ISSN 0016-7037. Retrieved 2015-02-04.
- ^ a b c Bostrom, C.-E.; Gerde, P.; Hanberg, A.; Jernstrom, B.; Johansson, C.; Kyrklund, T.; Rannug, A.; Tornqvist, M.; Victorin, K.; Westerholm, R. (2002). "Cancer risk assessment, indicators, and guidelines for polycyclic aromatic hydrocarbons in the ambient air". Environmental Health Perspectives. 110 (Suppl 3): 451–488. doi:10.1289/ehp.110-1241197. ISSN 0091-6765. PMC 1241197. PMID 12060843.
- ^ Loeb, L. A.; Harris, C. C. (2008). "Advances in Chemical Carcinogenesis: A Historical Review and Prospective". Cancer Research. 68 (17): 6863–6872. doi:10.1158/0008-5472.CAN-08-2852. ISSN 0008-5472. PMC 2583449. PMID 18757397.
- ^ a b c d e Dipple, A. (1985). "Polycyclic Aromatic Hydrocarbon Carcinogenesis". Polycyclic Hydrocarbons and Carcinogenesis. ACS Symposium Series. Vol. 283. American Chemical Society. pp. 1–17. doi:10.1021/bk-1985-0283.ch001. ISBN 0-8412-0924-3. Retrieved 2015-05-06.
- ^ International Agency for Research on Cancer (1984). Polynuclear Aromatic Compounds, Part 3, Industrial Exposures in Aluminium Production, Coal Gasification, Coke Production, and Iron and Steel Founding (Report). IARC Monographs on the Evaluation of Carcinogenic Risks to Humans. Lyon, France: World Health Organization. pp. 89–92, 118–124. Retrieved 2016-02-13.
- ^ a b c Baird, W. M.; Hooven, L. A.; Mahadevan, B. (2005). "Carcinogenic polycyclic aromatic hydrocarbon-DNA adducts and mechanism of action". Environmental and Molecular Mutagenesis. 45 (2–3): 106–114. doi:10.1002/em.20095. ISSN 1098-2280. PMID 15688365. S2CID 4847912. Retrieved 2014.
{{cite journal}}
: Check date values in:|accessdate=
(help) - ^ Slaga, T.J. (1984). "Chapter 7: Multistage skin carcinogenesis: A useful model for the study of the chemoprevention of cancer". Acta Pharmacologica et Toxicologica. 55 (S2): 107–124. doi:10.1111/j.1600-0773.1984.tb02485.x. ISSN 1600-0773. PMID 6385617. Retrieved 2015-05-06.
- ^ a b c Xue, W; Warshawsky, D (2005). "Metabolic activation of polycyclic and heterocyclic aromatic hydrocarbons and DNA damage: A review". Toxicology and Applied Pharmacology. 206 (1): 73–93. doi:10.1016/j.taap.2004.11.006. ISSN 0041-008X. PMID 15963346. Retrieved 2014-08-20.
- ^ Shimada, T; Fujii-Kuriyama, Y (2004-01-01). "Metabolic activation of polycyclic aromatic hydrocarbons to carcinogens by cytochromes P450 1A1 and1B1". Cancer Science. 95 (1): 1–6. doi:10.1111/j.1349-7006.2004.tb03162.x. ISSN 1349-7006. PMID 14720319. S2CID 26021902. Retrieved 2014-10-24.
- ^ Androutsopoulos, V.P.; Tsatsakis, A.M.; Spandidos, D.A. (2009). "Cytochrome P450 CYP1A1: wider roles in cancer progression and prevention". BMC Cancer. 9 (1): 187. doi:10.1186/1471-2407-9-187. ISSN 1471-2407. PMC 2703651. PMID 19531241.
{{cite journal}}
: CS1 maint: unflagged free DOI (link) - ^ a b Henkler, F; Stolpmann, K; Luch, Andreas (2012). "Exposure to Polycyclic Aromatic Hydrocarbons: Bulky DNA Adducts and Cellular Responses". In A. Luch (ed.) (ed.). Molecular, Clinical and Environmental Toxicology. Experientia Supplementum. Vol. 101. Springer Basel. pp. 107–131. doi:10.1007/978-3-7643-8340-4_5. ISBN 978-3-7643-8340-4. PMID 22945568. Retrieved 2015-04-03.
{{cite book}}
:|editor=
has generic name (help) - ^ a b c Nebert, D. W.; Dalton, T. P.; Okey, A. B.; Gonzalez, F. J. (2004). "Role of Aryl Hydrocarbon Receptor-mediated Induction of the CYP1 Enzymes in Environmental Toxicity and Cancer". Journal of Biological Chemistry. 279 (23): 23847–23850. doi:10.1074/jbc.R400004200. ISSN 1083-351X. PMID 15028720. Retrieved 2014-08-20.
- ^ a b Ramesh, A.; Walker, S. A.; Hood, D. B.; Guillén, M. D.; Schneider, K.; Weyand, E. H. (2004). "Bioavailability and risk assessment of orally ingested polycyclic aromatic hydrocarbons". International Journal of Toxicology. 23 (5): 301–333. doi:10.1080/10915810490517063. ISSN 1092-874X. PMID 15513831. S2CID 41215420. Retrieved 2015-04-13.
- ^ Korashy, H. M.; El-Kadi, A. O. S. (2006). "The Role of Aryl Hydrocarbon Receptor in the Pathogenesis of Cardiovascular Diseases". Drug Metabolism Reviews. 38 (3): 411–450. doi:10.1080/03602530600632063. ISSN 0360-2532. PMID 16877260. S2CID 30406435. Retrieved 2014-08-26.
- ^ a b Lewtas, J. (2007). "Air pollution combustion emissions: Characterization of causative agents and mechanisms associated with cancer, reproductive, and cardiovascular effects". Mutation Research/Reviews in Mutation Research. The Sources and Potential Hazards of Mutagens in Complex Environmental Matrices - Part II. 636 (1–3): 95–133. doi:10.1016/j.mrrev.2007.08.003. ISSN 1383-5742. PMID 17951105. Retrieved 2014-08-12.
- ^ a b c d Ramos, Kenneth S.; Moorthy, Bhagavatula (2005). "Bioactivation of Polycyclic Aromatic Hydrocarbon Carcinogens within the vascular Wall: Implications for Human Atherogenesis". Drug Metabolism Reviews. 37 (4): 595–610. doi:10.1080/03602530500251253. ISSN 0360-2532. PMID 16393887. S2CID 25713047. Retrieved 2015-03-27.
- ^ Kunzli, N.; Tager, I. (2005). "Air pollution: from lung to heart" (PDF). Swiss Medical Weekly. 135 (47–48): 697–702. PMID 16511705. Retrieved 2015-12-16.
- ^ Ridker, P. M. (2009). "C-Reactive Protein: Eighty Years from Discovery to Emergence as a Major Risk Marker for Cardiovascular Disease". Clinical Chemistry. 55 (2): 209–215. doi:10.1373/clinchem.2008.119214. ISSN 1530-8561. PMID 19095723. Retrieved 2015-12-16.
- ^ Rossner Jr, P.; Sram, R.J. (2012). "Immunochemical detection of oxidatively damaged DNA". Free Radical Research. 46 (4): 492–522. doi:10.3109/10715762.2011.632415. ISSN 1071-5762. PMID 22034834. S2CID 44543315. Retrieved 2015-12-17.
- ^ Sram, R.J.; Binkova, B.; Dejmek, J.; Bobak, M. (2005). "Ambient Air Pollution and Pregnancy Outcomes: A Review of the Literature". Environmental Health Perspectives. 113 (4): 375–382. doi:10.1289/ehp.6362. ISSN 0091-6765. PMC 1278474. PMID 15811825.
- ^ Winans, B; Humble, M; Lawrence, B. P. (2011). "Environmental toxicants and the developing immune system: A missing link in the global battle against infectious disease?". Reproductive Toxicology. 31 (3): 327–336. doi:10.1016/j.reprotox.2010.09.004. PMC 3033466. PMID 20851760.
- ^ Wormley, D D; Ramesh, A; Hood, D B (2004). "Environmental contaminant–mixture effects on CNS development, plasticity, and behavior". Toxicology and Applied Pharmacology. 197 (1): 49–65. doi:10.1016/j.taap.2004.01.016. ISSN 0041-008X. PMID 15126074. Retrieved 2014-09-12.
- ^ Suades-González, E; Gascon, M; Guxens, M; Sunyer, J (2015). "Air Pollution and Neuropsychological Development: A Review of the Latest Evidence". Endocrinology. 156 (10): 3473–3482. doi:10.1210/en.2015-1403. ISSN 0013-7227. PMC 4588818. PMID 26241071. Retrieved 2016-01-13.
- ^ a b c d Billiard, S. M.; Meyer, J. N.; Wassenberg, D. M.; Hodson, P. V.; Di Giulio, R. T. (2008). "Nonadditive effects of PAHs on Early Vertebrate Development: mechanisms and implications for risk assessment". Toxicological Sciences. 105 (1): 5–23. doi:10.1093/toxsci/kfm303. ISSN 1096-0929. PMC 2734299. PMID 18156145. Retrieved 2015-04-01.
- ^ a b c Reynaud, S.; Deschaux, P. (2006). "The effects of polycyclic aromatic hydrocarbons on the immune system of fish: A review". Aquatic Toxicology. 77 (2): 229–238. doi:10.1016/j.aquatox.2005.10.018. ISSN 0166-445X. PMID 16380172. Retrieved 2015-03-31.